Fine powders and granular materials are used extensively in industrial applications. These materials have to be accurately characterized to control as well as improve the processing techniques.
The characterization techniques are related to the grains’ properties like granulometry, chemical composition, morphology, and so on, or to the bulk powder behavior like density, flowability, electrostatic properties, blend stability, etc.
Conversely, with regards to the physical behavior of bulk powder, the majority of the methods applied in research and development and quality control laboratories are based on traditional measurement methods. Over the last 10 years, these methods have been scaled up to fulfill the current needs of production departments and research and development laboratories. Especially, the measurement processes have been automatized and stringent initialization techniques have been created to achieve results that can be reproduced and interpreted. Furthermore, the precision of the measurements is improved using image analysis methods.
A wide range of measurement techniques has been developed to meet all the requirements of industries processing granular materials and powders. Conversely, only the GranuCharge and GranuDrum instruments will be presented in this article:
- GranuCharge for measuring the electrostatic properties of powders
- GranuDrum for measuring flowing properties, for example, dynamic cohesive index, powder aeration, flowing angle, and first avalanche angle
GranuDrum
The GranuDrum instrument serves as an automated powder flowability measurement technique based on the principle of the rotating drum. A horizontal cylinder containing transparent sidewalls known as the drum is half filled with the powder sample. The drum spins around its axis at an angular velocity ranging between 2 and 60 rpm. Snapshots (30–100 images separated by 1 second) for each angular velocity are captured by a CCD camera.
An edge detection algorithm is used for detecting the air/powder interface on individual snapshots, and the average interface position and the variations around this average position are subsequently calculated. Next, the flowing angle αf — also called the “dynamic angle of repose” in the literature — for each rotating speed is calculated from the average interface position, and the dynamic cohesive index σf is determined from the interface fluctuations.
Generally, a low value of the flowing angle αf relates to a good flowability. A broad set of parameters — such as the shape of the grains, the friction between the grains, and the cohesive forces (electrostatic, capillary, and van der Waals forces) between the grains — influences the flowing angle. The dynamic cohesive index σf is only associated with the cohesive forces present between the grains. In addition, a non-cohesive powder leads to a regular flow, while a cohesive powder leads to an intermittent flow. As a consequence, a dynamic cohesive index close to zero relates to a non-cohesive powder. Increased cohesiveness of the powder translates to increased cohesive index.
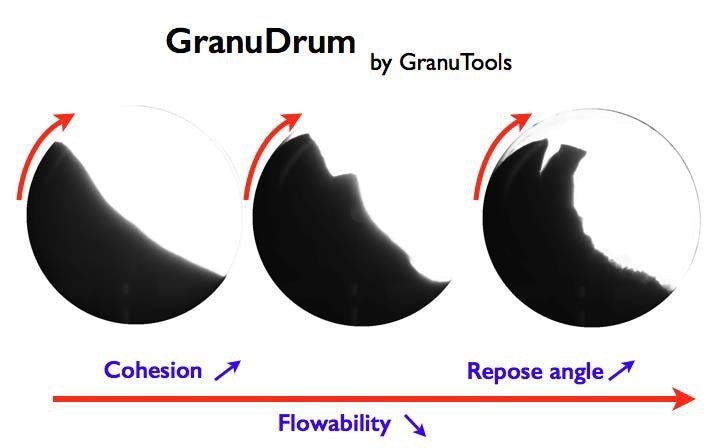
Apart from the measurement of the flowing angle αf as well as the cohesive index σf as a function of the rotating speed, the GranuDrum instrument enables measuring the powder aeration and the first avalanche angle at the time of the flow.
GranuCharge
During a flow, electrostatic charges are generated inside a powder. This apparition of electric charges is caused by the triboelectric effect, in which charge exchange occurs at the contact between two solids. When a powder flows inside a device (such as a conveyor, silo, mixer, and so on), the triboelectric effect occurs at the contact between the device and the grains and also at the contact between the grains. Therefore, the properties of the powder as well as the nature of the material used for building the device are said to be crucial parameters.
The GranuCharge instrument is designed to precisely and automatically measure the quantity of electrostatic charges generated within a powder during a flow in contact with a chosen material.
After the powder sample flows inside a vibrating V-tube, it falls in a Faraday cup linked to an electrometer, which, in turn, determines the charge obtained by the powder at the time of the flow within the V-tube. To achieve reproducible results, either a vibrating or a rotating device is used for feeding the V-tube regularly.
Selected Powders
During this study, a stainless-steel powder supplied by Höganäs company was used.
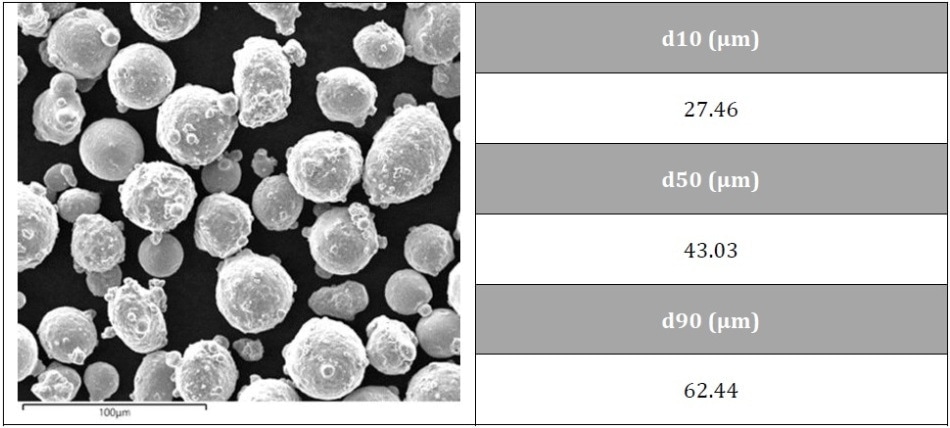
Figure 1. SEM picture and particle size distribution of the SS 316L powder provided by Höganäs.
This powder is available in two different versions — the original one supplied by the supplier, and a recycled one acquired following a selective laser melting (SLM) process in a 3D printer.
These powders, however, were kept in Granutools’ laboratory for a number of months. There, the samples’ history is not known and their properties could not relate to the producers’ specifications for fresh powders.
GranuDrum Analysis
Experimental Protocol
To perform an experiment with the GranuDrum instrument, powders were introduced inside the measuring cell soon after opening the box. The amount of powder used was about 50 mL. Each powder was examined under standard conditions (21 °C and 23% RH). Next, 20 GranuDrum velocities were analyzed (from 2 to 60 rpm) and for every velocity, 50 pictures were taken to boost the measurement’s repeatability and accuracy.
Experimental Results
The cohesive index and the dynamic angle of repose as a function of the GranuDrum rotating speed are shown in Figure 2. All the presented measurements were executed by increasing the speed of the drum and then by decreasing it (the main objective of this step is to emphasize a granulation phenomenon, that is, a thixotropic behavior). Powder flowability is quantified by the dynamic angle of repose (it considers the three cohesive forces: capillary, electrostatic, and Van der Waals, and also the contact forces between grains). The cohesive index — associated with the variations of the interface (air/powder) position — only denotes the three contact forces. Therefore, powder flowability is characterized by the dynamic angle of repose, while powder spreadability is quantified by the cohesive index.
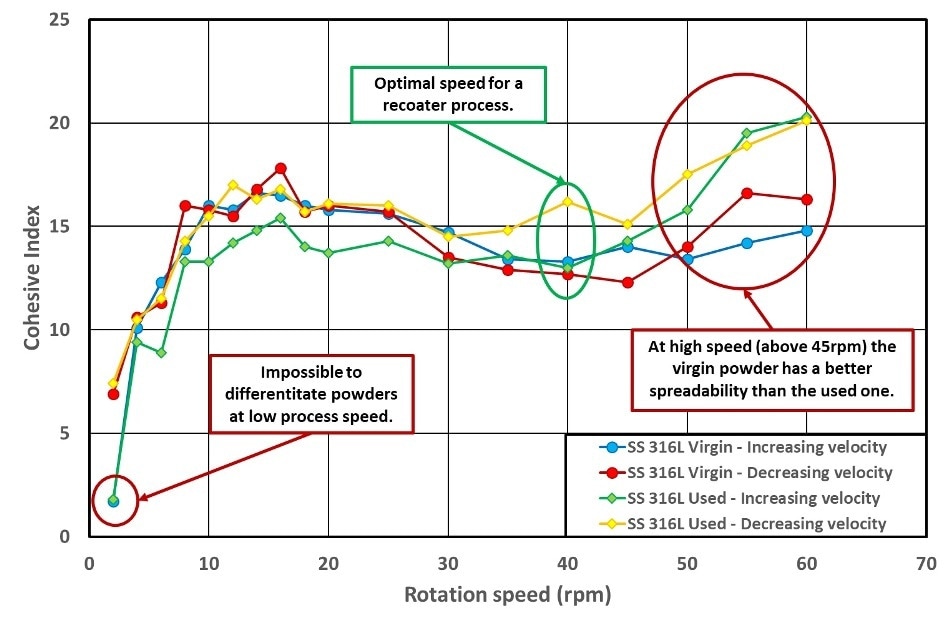
Figure 2. Cohesive index versus rotating drum speed.
Discussion
First, no thixotropic behavior can be emphasized (by decreasing or increasing the speed of the drum). Therefore, these samples are impervious to agglomeration. Powder spreadability — due to its original algorithm images treatment, that is, the cohesive index — can be obtained with excellent precision (1.8%). Indeed, for high velocities (above 45 rpm, that is, 200 mm/second, refer to Appendix 1 for speed conversion), the cohesive index of the virgin powder is seen to be lower compared to the used sample; therefore, it is better to apply the original powder for a recoater/SLM process instead of applying the used powder (G. Yablokova et al., 2015).
In addition, Figure 2 helps to optimize the process speed. Indeed, if a good spreadability has to be achieved at high speed, it can be inferred that a recoater speed of up to 170 mm/second is the perfect option because the cohesive index is satisfactory and the process speed is adequately high.
Figure 3 informs about powders flowability. Firstly, less than 20 rpm makes it difficult to differentiate between one powder to another, and this observation could be attributed to the similar flowing behavior exhibited by these powders. Secondly, above 20 rpm makes it possible to infer that the virgin powder’s flowability is better compared to the used one. Indeed, at 50 rpm, the dynamic angle of repose of the virgin powder is close to 60°, whereas it is equal to 65° in the case of the used sample.
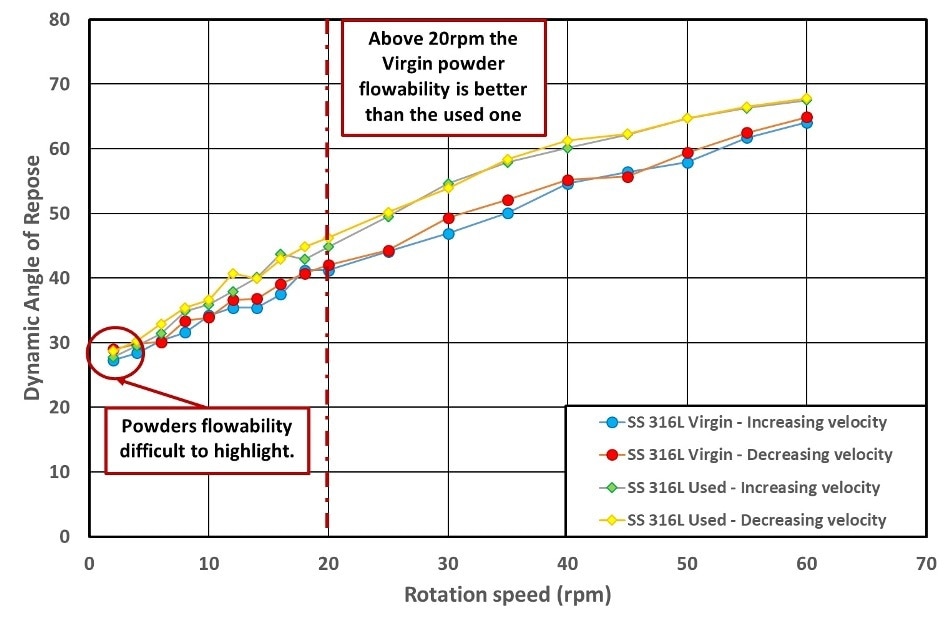
Figure 3. Dynamic angle of repose versus rotating drum speed.
Finally, the triboelectric effect is one potential explanation for the spreadability/flowability variations between the used and virgin powders. It is certainly possible that the used powder is more vulnerable to electrical charges, particularly at high speed. Therefore, to confirm this theory, a number of tests were performed with the GranuCharge instrument.
Carbon, Sulfur, Oxygen, and Nitrogen Content Analysis
Materials and Methods
Using the CS744 and ONH836 Elemental Analyzers, the LECO center (LECO European Application and Technology Center, 10589 Berlin, Germany) measured the carbon, sulfur, nitrogen, and oxygen content of the samples. The entire measurements were repeated thrice (n = 3); approximately 1 g was the typical sample mass.
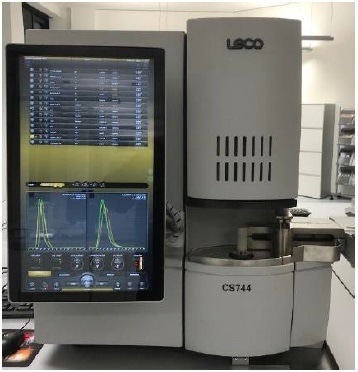
Figure 4. Photography of the CS744 analyzer.
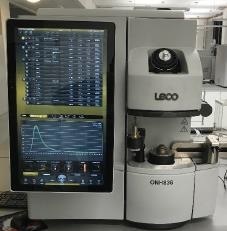
Figure 5. Photography of the ONH836 analyzer.
Experimental Results
The below figures show the oxygen/nitrogen content and carbon/sulfur content measured for the recycled and virgin stainless-steel powders. Also shown is the standard deviation.
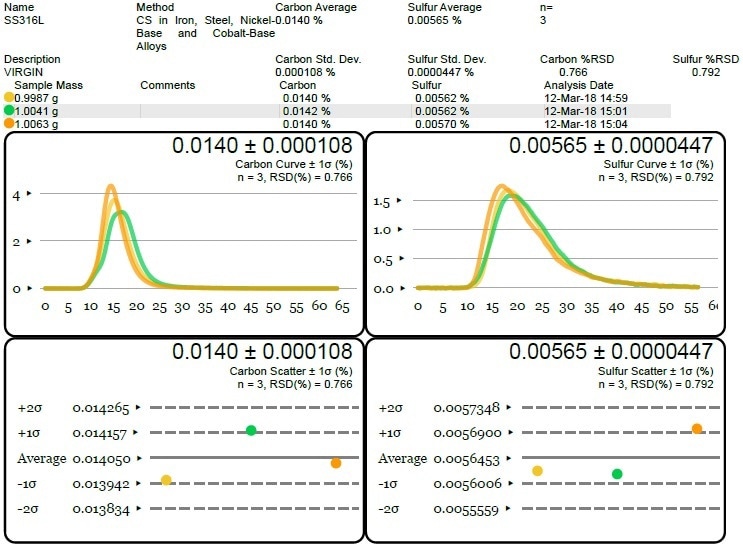
Figure 6. Carbon and sulfur content of the virgin SS 316L powder - CS744 analysis.
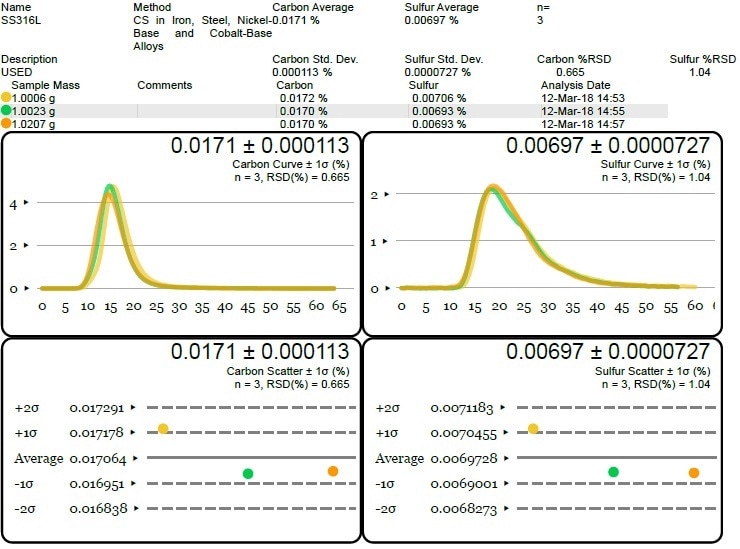
Figure 7. Carbon and sulfur content of the used SS 316L powder - CS744 analysis.
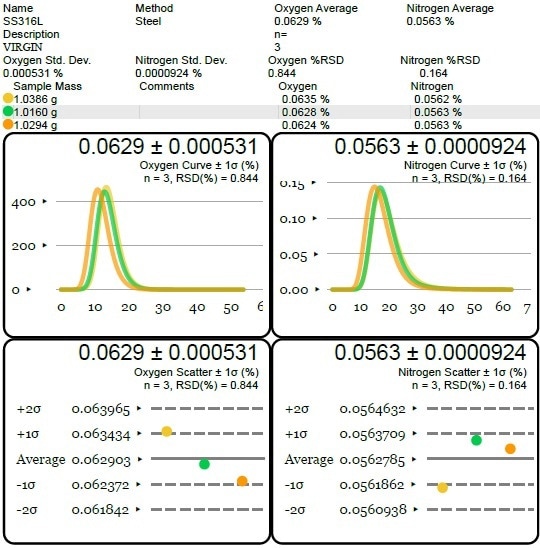
Figure 8. Oxygen and nitrogen content of the virgin SS 316L powder - ONH836 analysis.
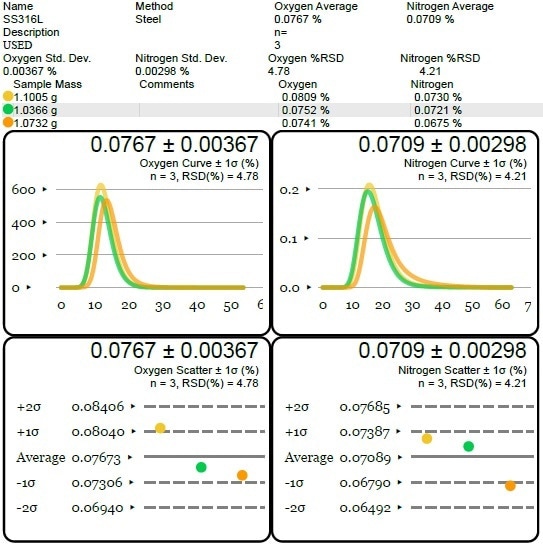
Figure 9. Oxygen and nitrogen content of the used SS 316L powder - ONH836 analysis.
Discussion
The following table summarizes the LECO results.
Table 1. Summary of the carbon/sulfur analysis for the virgin and used SS 316L powders
Sample Name |
Type of analysis |
Content ± σ (%) |
SS 316L Virgin Powder |
Carbon content |
0.01400 ± 0.00011 |
SS 316L Used Powder |
0.01710 ± 0.00012 |
SS 316L Virgin Powder |
Sulfur content |
0.00565 ± 0.00005 |
SS 316L Used Powder |
0.00697 ± 0.00008 |
SS 316L Virgin Powder |
Oxygen content |
0.0629 ± 0.0006 |
SS 316L Used Powder |
0.0767 ± 0.0037 |
SS 316L Virgin Powder |
Nitrogen content |
0.0563 ± 0.0001 |
SS 316L Used Powder |
0.0709 ± 0.0030 |
The analysis of carbon and sulfur is quite interesting, emphasizing that the SLM process slightly alters the powders’ composition. It can be seen that the sulfur and carbon content is more significant for the used powder (S = 0.00697% and C = 0.017%) when compared to the virgin one (S = 0.00565% and C = 0.014%). Furthermore, the nitrogen and oxygen content of the used powder (N = 0.0709% and O = 0.0767%) is also more significant compared to the virgin one (N = 0.0563% and O = 0.0629%). These are interesting observations, and regardless of the fact that the SLM is performed under an inert atmosphere, powders oxidation cannot be prevented.
GranuCharge Analysis
Experimental Protocol
The powders’ triboelectric effect was analyzed using the GranuCharge instrument. For every experiment performed with the GranuCharge instrument, rotating feeder and aluminum/stainless-steel 316L pipes were used (cf. Figure 10).
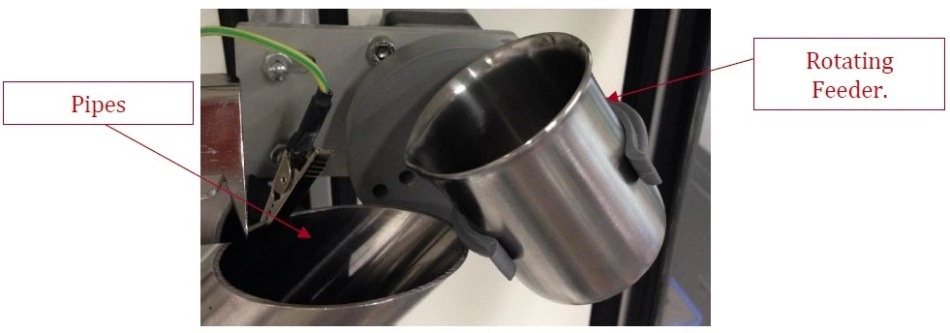
Figure 10. Photography of the rotating feeder used during the tests.
For each measurement, 50 mL of powder was used and the same was not reused after a measurement. Tests were again performed four times, followed by plotting the average value. All powders were examined under standard conditions (26 °C and 38% RH).
At the start of the test, the powder is introduced inside the Faraday cup to measure the initial powder charge density (qi, in nC/g). Upon completing this step, the powder is transferred inside the rotating feeder, and the experiment is then started. The end charge density is determined (qf, nC/g) toward the end of the experiment.
All the results achieved with the GranuCharge instrument are summarized in Table 2. Each charge density value relates to the average value computed between the four tests. (Δq = qfMean − q0Mean, in μC/kg, and σ is associated with the standard deviation acquired for each test).
Table 2. Synthesis of the results obtained with the GranuCharge instrument
Sample Name |
Pipes material |
q0 (nC/kg) |
qf (nC/kg) |
σqf |
Δq (nC/g) |
σΔq (nC/g) |
SS 316L Virgin Powder |
Aluminum |
-0.017 |
-0.069 |
0.004 |
-0.052 |
0.004 |
SS 316L Used Powder |
-0.011 |
-0.050 |
0.004 |
-0.039 |
0.004 |
SS 316L Virgin Powder |
SS 316L |
-0.017 |
-0.127 |
0.004 |
-0.110 |
0.004 |
SS 316L Used Powder |
-0.011 |
-0.105 |
0.004 |
-0.094 |
0.004 |
Results Interpretation
Figure 11 shows that using the GranuCharge instrument, differentiation between powders can be made with excellent precision (best accuracy is 3.4%), which is indeed interesting. Even the initial charge densities are seen to be considerably different from one powder to another. Obviously, initial charge density for the virgin powder is the highest (–0.017 nC/g), while this is the lowest for the recycled sample, with a charge density equal to –0.011 nC/g. The charges densities of the samples are negative, and hence, they are anionic powders.
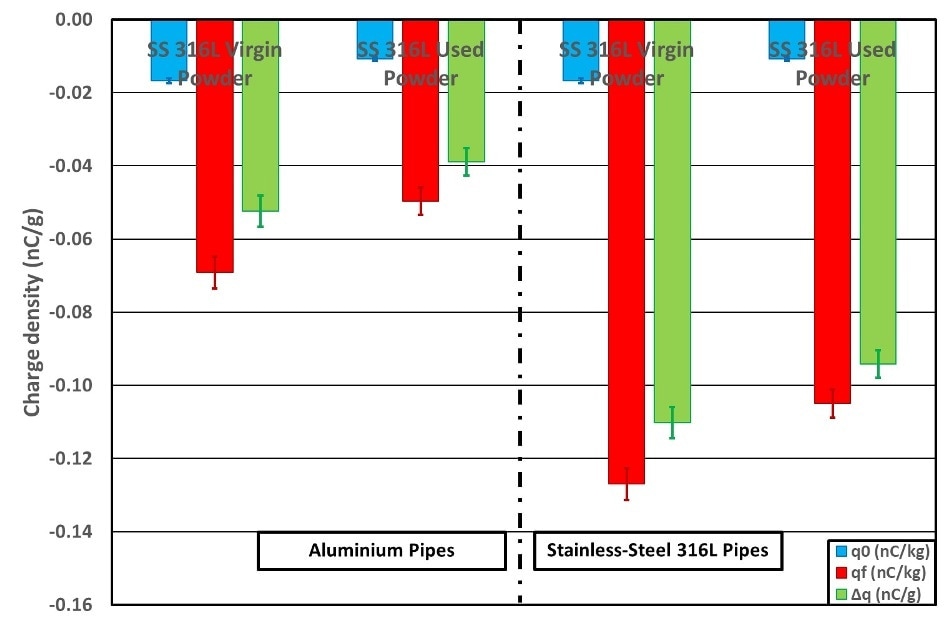
Figure 11. Histogram of the comparison between the initial and final charge densities for SS 316L powders.
After the powders flow, making contact with stainless-steel 316L and aluminum pipes, the electrical charges built up by them are also negatives. Yet, regardless of the type of pipe material used, the build-up of electrical charge is higher for the virgin powder compared to the used one. Therefore, the virgin sample is more vulnerable to the triboelectric effect.
The Carbone analysis may give one potential explanation for these observations. Compared to the virgin powder, the carbon content is indeed higher for the used one. In addition, carbon is known as an anti-static agent, and hence, increased powder carbon content may not only lead to a reduction in the initial electrical charge but may also result in the build-up of a lower charge density after a flow.
Conversely, the previous conclusion has to be carefully considered. Obviously, the recycling process post an SLM operation may cause a number of complex changes in the physicochemical properties of the powder. Such changes have particles size distribution/shape, and also surface characteristics may occur and make it difficult to interpret results.
Conclusions
- Metallic powder aging for AM is difficult to emphasize with traditional techniques, like Densi-Tap and Hall flowmeter, because of their user dependency and substandard reproducibility.
- Conversely, with the LECO and Granutools instruments, the recycling after an SLM process can be followed with excellent precision.
- Using the GranuDrum instrument, conclusions can be reached related to the flowability and spreadability of a virgin powder and a used SS 316L powder (close to 2% accuracy). The flowability and spreadability of the virgin powder have been shown to be better when compared to those of the used one.
- Through the GranuCharge analysis, the virgin powder is shown to be more sensitive to electrical charge (3.4% is the best-achieved accuracy). Yet, the use of aluminum as a process material can reduce the build-up of electrical charges, particularly with a recoater as a way to disperse the powder.
- The GranuCharge results seem to be confirmed by the LECO analysis with the carbon, sulfur, nitrogen, and oxygen contents (CS 744 and ONH 836 instruments). Based on these measurements, it can be said that the carbon, sulfur, nitrogen, and oxygen contents are higher for the recycled powder compared to the virgin one. As an anti-static agent, carbon can be one potential explanation about the GranuCharge analysis.
Appendix
Appendix 1: Relation Between Drum Rotating Speed and Process Speed (in mm/ second)
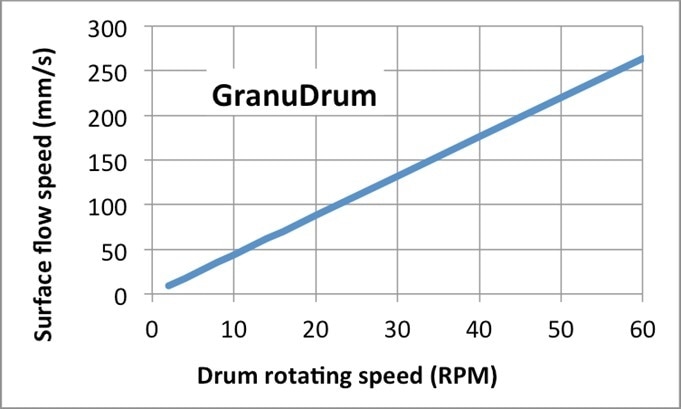
Figure 12. Relation between drum rotating speed and process speed (in mm/second).
Bibliography
- Cascade of granular flows for characterizing segregation, G. Lumay, F. Boschin, R. Cloots, N. Vandewalle, Powder Technology 234, 32-36 (2013).
- Combined effect of moisture and electrostatic charges on powder flow, A. Rescaglio, J. Schockmel, N. Vandewalle and G. Lumay, EPJ Web of Conferences 140, 13009 (2017).
- Compaction dynamics of a magnetized powder, G. Lumay, S. Dorbolo and N. Vandewalle, Physical Review E 80, 041302 (2009).
- Compaction of anisotropic granular materials: Experiments and simulations, G. Lumay and N. Vandewalle, Physical Review E 70, 051314 (2004).
- Compaction Dynamics of Wet Granular Assemblies, J. E. Fiscina, G. Lumay, F. Ludewig and N. Vandewalle, Physical Review Letters 105, 048001 (2010).
- Effect of an electric field on an intermittent granular flow, E. Mersch, G. Lumay, F. Boschini, and N. Vandewalle, Physical Review E 81, 041309 (2010).
- Effect of relative air humidity on the flowability of lactose powders, G. Lumay, K. Traina, F. Boschini, V. Delaval, A. Rescaglio, R. Cloots and N. Vandewalle, Journal of Drug Delivery Science and Technology 35, 207-212 (2016).
- Experimental Study of Granular Compaction Dynamics at Different Scales: Grain Mobility, Hexagonal Domains, and Packing Fraction, G. Lumay and N. Vandewalle, Physical Review Letters 95, 028002 (2005).
- Flow abilities of powders and granular materials evidenced from dynamical tap density measurement, K. Traina, R. Cloots, S. Bontempi, G. Lumay, N. Vandewalle and F. Boschini, Powder Technology, 235, 842-852 (2013).
- Flow of magnetized grains in a rotating drum, G. Lumay and N. Vandewalle, Physical Review E 82, 040301(R) (2010).
- How tribo-electric charges modify powder flowability, A. Rescaglio, J. Schockmel, F. Francqui, N. Vandewalle, and G. Lumay, Annual Transactions of The Nordic Rheology Society 25, 17-21 (2016).
- Influence of cohesives forces on the macroscopic properties of granular assemblies, G. Lumay, J. Fiscina, F. Ludewig and N. Vandewalle, AIP Conference Proceedings 1542, 995 (2013).
- Linking compaction dynamics to the flow properties of powders, G. Lumay, N. Vandewalle, C. Bodson, L. Delattre and O. Gerasimov, Applied Physics Letters 89, 093505 (2006).
- Linking flowability and granulometry of lactose powders, F. Boschini, V. Delaval, K. Traina, N. Vandewalle, and G. Lumay, International Journal of Pharmaceutics 494, 312–320 (2015).
- Measuring the flowing properties of powders and grains, G. Lumay, F. Boschini, K. Traina, S. Bontempi, J.-C. Remy, R. Cloots, and N. Vandewall, Powder Technology 224, 19-27 (2012).
- Motion of carbon nanotubes in a rotating drum: The dynamic angle of repose and a bed behavior diagram, S. L. Pirard, G. Lumay, N. Vandewalle, J-P. Pirard, Chemical Engineering Journal 146, 143-147 (2009).
- Mullite coatings on ceramic substrates: Stabilisation of Al2O3–SiO2 suspensions for spray drying of composite granules suitable for reactive plasma spraying, A. Schrijnemakers, S. André, G. Lumay, N. Vandewalle, F. Boschini, R. Cloots and B. Vertruyen, Journal of the European Ceramic Society 29, 2169–2175 (2009).
- Rheological behavior of β-Ti and NiTi powders produced by atomization for SLM production of open porous orthopedic implants, G. Yablokova, M. Speirs, J. Van Humbeeck, J.-P. Kruth, J. Schrooten, R. Cloots, F. Boschini, G. Lumay, J. Luyten, Powder Technology 283, 199–209 (2015).
- The flow rate of granular materials through an orifice, C. Mankoc, A. Janda, R. Arévalo, J. M. Pastor, I. Zuriguel, A. Garcimartín and D. Maza, Granular Matter 9, p407–414 (2007).
- The influence of grain shape, friction and cohesion on granular compaction dynamics, N. Vandewalle, G. Lumay, O. Gerasimov and F. Ludewig, The European Physical Journal E (2007).
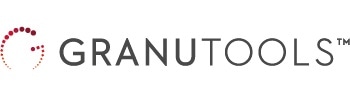
This information has been sourced, reviewed and adapted from materials provided by Granutools.
For more information on this source, please visit Granutools.