Vesicles, micelles and microemulsions aroused considerable attention during the advent of what would become dynamic light scattering.1 This took place in the 1970s, prior to the invention of the term nanotechnology and the first biotech revolution.
However, it was not until the 1990s that DLS instruments were small enough, powerful enough, and automated enough to be of routine utility for these proposed targeted drug delivery systems.
Liposomes are synthetic versions of natural vesicles such as exosomes, microvesicles, ectosomes, prostasomes, and several others that are characterized by their biogenesis.2 Liposomes represented a hot topic in the 1980s and 1990s, when it was believed that they could be leading candidates for targeted drug delivery.
However, as they are not natural to the body, it was found that they were attacked by white blood cells and cut open, causing their relatively high dosage of internal drugs to spill into the blood system, typically at a distance from the targeted (cancerous, for example) organ.
Although there have been some advances brought about with the addition of surface moieties, which deceive the white blood cells, it is unclear if this can genuinely become a significant contender for targeted drug delivery.
Microvesicles, liposomes, and exosomes are core-shell particles. The core is water with drugs (liposomes) or cytoplasmic proteins, small RNAs, cell-specific receptors, and surface markers (exosomes and microvesicles). The shell is a lipid layer. The liposomes, which are artificial and lack the natural markers to deceive white blood cells, were attacked.
Table 1. Properties and Origins
Name |
Properties |
Origin |
Exosome |
Homogeneous, 40-100 nm diameter, Exosomal surface markers CD9, CD63, Alix, flotillin-1, etc. |
Release from cells when multi-vesicular bodies fuse with the plasma membrane |
Micro vesicles |
Heterogeneous, 50-1,000 nm diameter. No unique markers |
Fission directly from the plasma membrane |
Liposomes |
Homogeneous, 50-250 nm diameter. Some with surface chemistry, many without |
Synthetically made. No natural defense in bodily fluids. |
Particle Sizing
A sample initially generated from FBS (fetal bovine serum) was prepared and diluted in PBS at pH 7.4. A 50 uL disposable BI-SM50 cell was utilized and placed into the Brookhaven 90Plus. The temperature was established at 25°C and equilibration were permitted for a number of minutes. Three runs of three minutes were undertaken at a 90° scattering angle, all yielding similar results to within a few percent.
The Effective Diameter measured 131.5 nm and the PDI (polydispersity index) measured 0.208. A size over 100 nm powerfully indicates polydispersity, while a PDI of 0.025 or less represents a narrow size distribution. The intensity-, volume-, and number-weighted size distributions were computed. As demonstrated in the graphs, the results indicate a bimodal size distribution, with one mode around 80nm and the other three times larger around 240 nm, rather than a broad unimodal distribution.
It is commonly recognized that a relatively low number of large particles will dominate the intensity-weighted size distribution, while also shifting the Effective Diameter higher.
However, the percentage contribution made by the smaller particles increases when the distribution is recast as volume-weighted. Indeed, the smaller particles can easily dominate when the distribution is further recast, to compare to number counting instruments such as electron microscopes. In this instance, after rounding to the nearest 0.5%, there is apparently no significant contribution made by the larger particles, while the remaining 80nm particles occur in the expected exosome size range.
Sizing Summary
Due to the fact that microvesicles and exosomes are additional means of transferring genetic material between cells, they are contenders for a further attempt at targeted drug delivery, as well as for research into the transfer of pathogenic proteins and RNAs. The ability to examine their size and state of aggregation rapidly and repeatedly utilizing DLS assists in the characterization of their properties. This application note explains that capacity.
.jpg)
Zeta Potential
Charges at the surface of a particle in a liquid configured a distribution of charge density around the particle. This causes electrostatic potential differences, one of which is referred to as zeta potential. If the zeta potential is too close to zero, there is an absence of electrostatic repulsion between particles and consequently the always-present, naturally occurring attractive forces will cause particles to aggregate. This engenders instability. To maintain stability, electrostatically stabilized particles should embody a finite zeta potential, usually more than 20 to 30 millivolts (mV).
Nevertheless, due to the fact that zeta potential is also dependent on the free salt ion concentration, the zeta potential can be lower in a high salt concentration environment and while retaining enough repulsion to separate the particles. Zeta potential is computed from the determination of electrophoretic mobility: charged particles in the liquid between electrodes move following the application of a field.
Measuring this movement is very complex in high salt, primarily because the current is often too high, c heating and chemical changes. Measuring in physiological saline or PBS are especially complex for this reason. The Brookhaven ZetaPALS applies low current in high salt and utilizes phase analysis light scattering to quantify with very small movements of charged particles with great sensitivity. As a result, it is uniquely suited to such complex measurements.
Sample preparation was identical as that described above under particle sizing. The electrodes are placed into a square plastic cell with around 1250 µL of suspension. The temperature was established at 25 °C and the sample equilibration was permitted for five minutes.
The average measured conductance was 27000 µS. Comparatively, conventional quantifications are performed from 1 to 10 millimolar of 1:1 electrolyte. These concentrations embody conductance spanning 320 to 3200 µS. Passing excessive current for excessive durations can cause irreparable changes to the sample and/or heat the liquid, either of which will effectively destroy the measurement of electrophoretic motion.
Consequently, measurements were taken at 1, 5, 10, 20, 30, 30, 30 and another 30 cycles, with a cycle corresponding to 1 second with the field on in one direction and another second where it is reversed, thus preventing the electrodes from polarizing. The cycle- weighted average of the initial 126 cycles was -17.7 mV, while, the average was -3.36 mV for the final 30 cycles. This indicates that the sample underwent an irreversible change as a result of the passage of excessive current.
Zeta Potential Summary
Quantifications in high salt such as saline or PBS are very complex. The Brookhaven ZetaPALS has been achieving this since the 1990s through the use of Phase Analysis Light Scattering. In this article, results deriving from an Exosome in PBS indicate a zeta potential of -17.7mV, which is perhaps insufficient for total stability, as demonstrated by the aggregates in size which were quantified, as well as the monomer size.
References and Further Reading
- Degiorgio, V., Corti, M., Giglio, M., editors, “Light Scattering in Liquids and Macromolecular Solutions”, 1979, Plenum Press, New York. See Section II, p. 111 on micelles, p. 125 on vesicles, and p. 139 on microemulsions.
- Lee, Y., El Andaloussi, S., Wood, M.J.A., Human Molecular Genetics, 2012, Vol. 21, Rev. Issue 1, R125- R134.
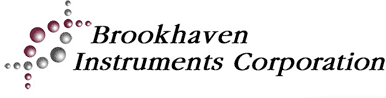
This information has been sourced, reviewed and adapted from materials provided by Brookhaven Instrument Corporation.
For more information on this source, please visit Brookhaven Instrument Corporation.