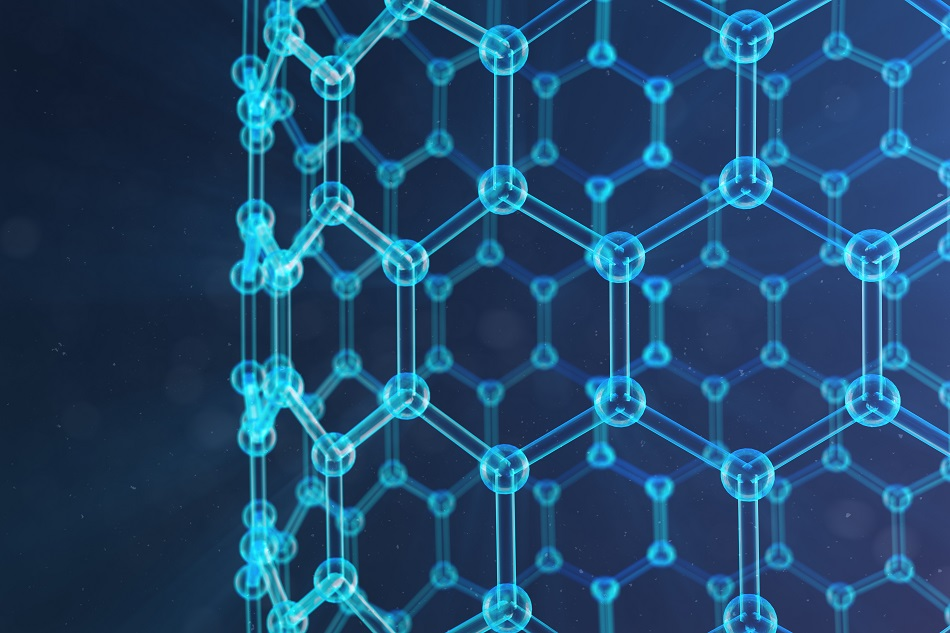
Image Credit: Rost9/Shutterstock.com
Graphene is an extraordinary material, the only basic member of a family of 2D carbon materials. With its unique mechanical and physical properties, it is a superb filler at the nanoscale, in metal matrix composite materials. It can easily disperse into the matrix and is cheaper than its closest competitor, carbon nanotubes, which enhance strength and function. The increased surface-to-volume ratio of graphene also means that its properties are better reflected in the composite.
Graphene alloys are popular because metals have good mechanical strength and elasticity, hardness, electrical conductivity, and thermal conductivity while graphene holds the world record for tensile strength at 130 GPa, about 200 times more than that of steel at 600 MPa.
The use of graphene to fill the matrix spaces is superior to oxide or carbide fillers which cause a loss of conductivity to heat and electricity. With graphene filler, there is an improvement in mechanical properties while retaining excellent heat and electrical attributes. The presence of even low loads of graphene allows the final material to be significantly reinforced concerning its mechanical properties.
Copper-Graphene Composites
Conventional copper alloys are widely used because they are excellent conductors of heat and electricity, and are chemically stable. Conversely, they are not very mechanically robust, particularly when heated. The best way to enhance the strength of copper and its alloys, and the only feasible way to improve their Young’s modulus, is to create copper matrix composites by introducing a secondary phase in these metals.
Copper-graphene composites were reported in 2013. Here, graphene particles are embedded within a copper matrix, or where graphene and copper are layered, as well as in configurations that mimic the nanolayered composite structure of mother-of-pearl.
Using tiny amounts of graphene at 0.00004% by weight, the superlative strength of graphene can be imparted to the composite with a fractional loading of graphene. The copper-graphene composite has a tensile strength of 1.5 GPa, which is half as strong again as titanium and 3 times more than that of aluminum alloys.
Cu-graphene composites can be fabricated with as much as 93% more hardness, 65% higher Young’s modulus, 230% more yield stress and 48% more tensile strength than copper. The hardness and other mechanical properties remain higher even at high temperatures.
Processing Methods and Cu-Graphene Composite Properties
The processing and fabrication techniques used have a pronounced effect on the properties of the final composite. Processing of graphene is complicated because of the need to produce a homogeneous dispersion of graphene within the matrix with a strong bond between the different molecules, as well as ensuring the structure of graphene remains unchanged throughout and after the processing. Some important techniques used in this area include powder metallurgy (PM), electrochemical deposition, and less common ones like chemical vapor deposition (CVD), cold spraying, layering assembly, metal infiltration, and preform impregnation.
With PM, graphene is mixed with raw metal powder to produce a composite which is then compacted into a bulk shape by densification using sintering, pressing, or rolling processes singly or in combination.
Simple mixing techniques by mechanical, magnetic, or sonication can be used to prepare the composite powder or more sophisticated ones like ball milling or mechanical alloying in the solid-state.
With electrochemical deposition, either via electroplating or electroless plating, the dispersion of graphene is improved. In the first, a source of electricity is used to deposit a film of composite on the cathode. This preserves graphene properties much better. With the second, the metal salt is decomposed by thermochemical means to release metal ions that form a composite with graphene, such as graphene-metal nanoparticles, sandwich nanocomposites of copper layers on either side of the graphene oxide.
Molecular-level mixing or PM has also been used to produce graphene decorated with silver or nickel nanoparticles to be used as fillers in copper matrices. CVD is a technique based on enveloping copper powders with graphene followed by compaction to bulk forms. This achieves bulk formation of composites, with improved dispersions and good bonding at the interfaces, while avoiding damage to the graphene structure as with other mechanical techniques. Another approach is to grow graphene on the surface of the copper powder by in situ CVD, as well as the creation of bioinspired nanolaminates by growing graphene on metal flakes that are then self-assembled and consolidated. Such structures may be produced by electrodeposition, electroless plating or thermochemical deposition.
Layer-by-layer assembly is slow but can produce a large variety of copper-graphene composite films. Metal infiltration uses melted metal introduced into a reinforcing preform to overcome insolubility barriers to the preparation of certain composites like tungsten-copper alloys. These produce high density as well as a homogeneous distribution but could damage the reinforcement, and cause unwanted reactions at the interface as well as graphene structural damage.
Other Graphene-Metal Composites
Tungsten-Copper
Some novel alloys potentially suitable for the addition of graphene include Cu-W (copper-tungsten) and W-Cu (tungsten-copper). These are metal-in-metal dispersions, called metal-matrix composites, rather than true alloys, because these do not dissolve in each other. When created in varying compositions, they show outstanding heat and electrical properties like that of copper, but the arc erosion resistance and low thermal expansion coefficient of tungsten. They promise to replace conventional Cu-W mixtures used in various electrical and welding applications but are unable to meet the evolving demands posed by newer electrical setups that need contact materials capable of sustaining ultrahigh voltages and large capacities.
Aluminum-Graphene
Graphene nanoflakes (GNFs) have also been used to reinforce aluminum alloys using PM, to produce a large increase in mechanical properties while retaining the characteristic ductility of aluminum and avoiding interfacial reactions.
Nickel-Graphene
Nickel (Ni) and Ni alloys are extremely strong and stable, used widely to meet application needs in high-temperature, extreme environments such as combustion engines or turbine blades. Nacre-like nickel-graphene composites have also been created using nickel powder and graphene sheets, with a resulting increase in strength of 73%, but a 28% reduction in ductility. Overall, the structure is much tougher than the original nickel carbide. The final graphene-derived Ni-Ti-Al/Ni3C kept its hardness even at high temperatures, up to 1000 °C. Nickel-graphene composites can have a tensile strength of about 4GPa that is 180 times that of raw nickel.
Others
Other metal matrix composites incorporated with graphene as the secondary phase includes magnesium, nickel, platinum, gold, cobalt, palladium, and silicon.
High-Entropy Alloys
In 2018, a high entropy alloy nanoparticle decorated graphene was reported. These multi-component alloys also referred to as high entropy alloys (HEAs) contain five or more different types of metal atoms in a solid solution held in a single-phase structure. The high configurational entropy holds these components in a solid solution rather than intermetallic compound formation. Such HEAs have extreme resistance to corrosion, wear, and oxidation, and are very strong.
The reason for such strength is the role played by graphene in preventing the slippage of the metal atoms from their locations. The ultrathin but ultrastrong graphene layers physically keep the metal atoms from sliding therefore preventing metal fracture. Such strong composites could be used in many ways, from automotive uses and aerospace designs, as well as in building structures and industrial processes.
Sources and Further Reading
- Anthony, S. (2013). Graphene used to make graphene-copper composite that’s 500 times stronger. https://www.extremetech.com/extreme/164961-graphene-used-to-make-graphene-copper-composite-thats-500-times-stronger
- Processing of graphene/CNT-metal powder. Prashantha Kumar HG and Anthony Xavior M. Powder Technology, Alberto Adriano Cavalheiro, IntechOpen, DOI: 10.5772/intechopen.76897. https://www.intechopen.com/books/powder-technology/processing-of-graphene-cnt-metal-powder
- First report on high entropy alloy nanoparticle decorated graphene. M. Y. Rekha, Nitin Mallik, and Chandan Srivastava. Scientific Reports 2018; 8: 8737. doi: 10.1038/s41598-018-27096-8. https://www.ncbi.nlm.nih.gov/pmc/articles/PMC5992158/
- Bioinspired, graphene-enabled Ni composites with high strength and toughness. Yunya Zhang, Frederick M. Heim, Jamison L. Bartlett, Ningning Song, Dieter Isheim, and Xiaodong Li. Science Advances 31 May 2019: Vol. 5, no. 5, eaav5577. DOI: 10.1126/sciadv.aav5577. https://advances.sciencemag.org/content/5/5/eaav5577.full
- Investigating aluminum alloy reinforced by graphene nanoflakes. S.J.Yan, S.L.Dai, X.Y.Zhang, C.Yang, Q.H.Hong, J.Z.Chen, and Z.M.Lin. Materials Science and Engineering. https://doi.org/10.1016/j.msea.2014.06.077. https://www.sciencedirect.com/science/article/abs/pii/S092150931400803X
- Copper/graphene composites: a review. Paloma Hidalgo-Manrique, Xianzhang Lei, Ruoyu Xu, Mingyu Zhou, Ian A. Kinloch & Robert J. Young. Journal of Materials Science volume 54, pages12236–12289(2019). https://doi.org/10.1007/s10853-019-03703-5. https://link.springer.com/article/10.1007/s10853-019-03703-5#Sec43
Disclaimer: The views expressed here are those of the author expressed in their private capacity and do not necessarily represent the views of AZoM.com Limited T/A AZoNetwork the owner and operator of this website. This disclaimer forms part of the Terms and conditions of use of this website.