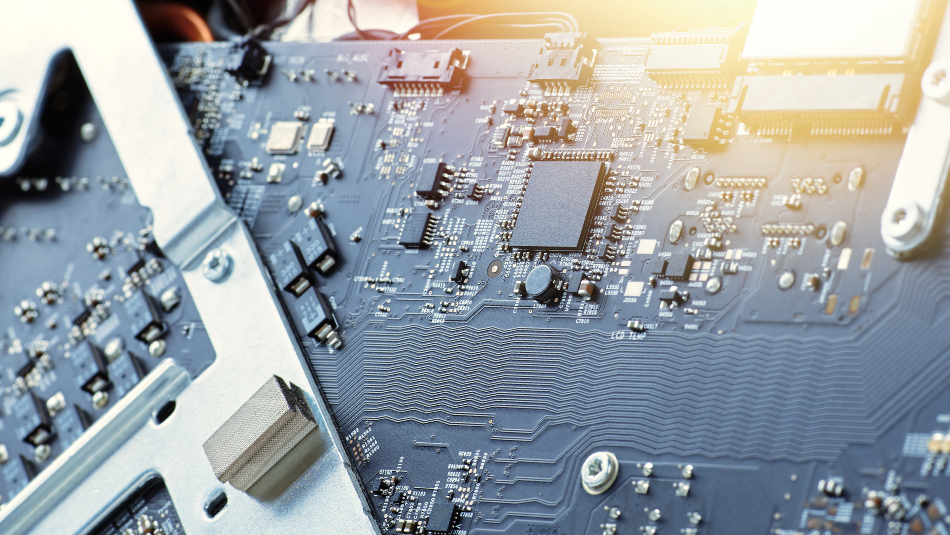
Image Credit: SergeyKlopotov/Shutterstock.com
Silicon chips control everything from washing machines to personal computers. Light emission has been hailed as the ‘Holy Grail’ of the microelectronics industry for decades. The speed of supercomputers could increase manifold if their chips could communicate by light rather than electricity. Solving the puzzle of producing silicon (Si) capable of light emission could help revolutionize the electronics industry.
Why are Photons Better than Electrons for Intra/Inter-Chip Communication?
Lower energy consumption
The resistance faced by electrons while traveling through the copper connections between transistors within a chip produces a lot of heat. In contrast, photons are packets of light without any mass or charge. They do not face any resistance and, therefore, no heat is produced. This greatly reduces energy consumption.
Higher rate of data transmission
Optical communication can speed up the intra- as well as inter-chip communication by a factor of 1000.
Why Si or Similar Semiconductor Variants Cannot Emit Light
When the momentum vectors (k) in a Brillouin zone representing a minimum-energy state in the conduction band and maximum-energy state in the valence band of a semiconductor are different, the material has an indirect gap.
The energy band gap is called direct if the k-vector is the same in both the conduction band and the valence band. In that case, an electron can emit a photon.
In an indirect gap, the electron cannot emit a photon because it has to pass through an intermediate state and transfer momentum to the crystal lattice in forms of phonons.
Even though cubic Si has dominated the electronics industry for more than 50 years, and is abundantly available as silicon oxide (SiO2), it, along with similar variants such as Germanium (Ge) and SiGe alloys, is an indirect bandgap semiconductor. Therefore, it cannot emit light efficiently.
What is the Application for Light Emission?
For optical transmission to happen within chips, A source of light is needed and an integrated laser.
However, although bulk Si is the most popular material in the semiconductor industry, it is inefficient at emitting light, and so is not considered a good candidate for photonics research.
Other materials such as Gallium Arsenide (GeAs) and Indium Phosphide (InP) have been explored. Even though these materials show good potential for light emission, they are more expensive than Si and are hard to integrate into existing Si microchips. To create a laser compatible with Si, a new form of Si that can emit light has been researched.
Previous Research
Previous research based on nanostructured silicon showed that the optoelectronic properties of silicon can be modified by quantum confinement effects.
Studies on porous silicon pioneered this approach and showed that, once silicon is reduced to nanometric dimensions, a bright red luminescence occurs at room temperature.
However, unless compatibility of porous silicon has been demonstrated for microelectronics and a demonstration of the integration of driving circuits with light-emitting element has been performed, factors such as the disordered distribution of nanocrystal sizes, inter-connectivities and surface reactivity to chemical agents are predicted to hamper a real-life application of porous silicon properties.
Further research predicted Si-nc embedded in amorphous silica (a-SiO2) to be comparatively better candidates for optoelectronics. Their robustness, stability and full compatibility with the mainstream CMOS technology induced this prediction.
The generation of visible light from Si-nc embedded in a-SiO2 matrix has been studied quite exhaustively to obtain optically tuneable quantum systems by modifying the nanoparticles’ dimensions [1].
Recent Developments
Researchers from the Eindhoven University of Technology, Netherlands, have recently developed an Si and Ge alloy with a hexagonal structure. It is claimed to feature a direct band-gap that allows light emission.
Band-folding leads to a direct band-gap being observed in hexagonal Ge at the high symmetry point Γ in the Brillouin Zone.
The researchers tried to tune direct band-gaps by alloying hexagonal Ge with Si. They carried out computation with a number of random compositions of the hex-Si(1−x)Gex alloy system and found a direct bandgap at the Γ-point for x > 0.65.
Click here to find out more about coating testing solutions
How can the Growth of this Alloy be Enforced?
When grown on planar hexagonal Ge, the cubic structure of Si remains unchanged.
The scientists at the Eindhoven University of Technology grew a Ge-rich Si(1−x)Gex alloy shell around a thin gold (Au with wurtzite structure) catalyzed GaAs core nanowire.
A thin GaAs core was used to reduce lattice strain and strain-induced defects. The catalytic particles of Au were then removed by wet chemical etching. This method enforces the growth of Si atoms in the hexagonal structure.
Future Research
If the light emission properties of Si can be tweaked using this approach, it would greatly benefit data institutes and centers by providing them with faster data transfer capabilities and reducing energy consumption for cooling.
The photonic chips produced with optical transmission capabilities will also bring about new applications. Some domains of possible applications include:
- Laser-based radars for self-driving cars
- Chemical sensors for medical diagnosis
- Food quality detection
- Air pollution detection.
However, the integration of this technology with existing ones is something that needs much more research to make this technology fully available commercially.
References and Further Reading
1. Vijay Kumar (2008) Nanosilicon. Elsevier Science. Available at: https://www.sciencedirect.com/book/9780080445281/nanosilicon
2. Fadaly, E.M.T., Dijkstra, A., Suckert, J.R. et al. (2020) Direct-bandgap emission from hexagonal Ge and SiGe alloys. Nature 580, 205–209. Available at: https://doi.org/10.1038/s41586-020-2150-y
3. Science Daily (2020) Revolutionary light-emitting silicon. [Online] Available at: https://www.sciencedaily.com/releases/2020/04/200408113250.htm (Accessed on 10 June 2020).
Disclaimer: The views expressed here are those of the author expressed in their private capacity and do not necessarily represent the views of AZoM.com Limited T/A AZoNetwork the owner and operator of this website. This disclaimer forms part of the Terms and conditions of use of this website.