Plastic constitutes a significant portion of the inorganic solid waste generated daily in municipal solid waste (MSW). The substantial volume of plastic waste has severe environmental repercussions, contributing to soil pollution through landfilling, water pollution when dumped into oceans, and air pollution.1
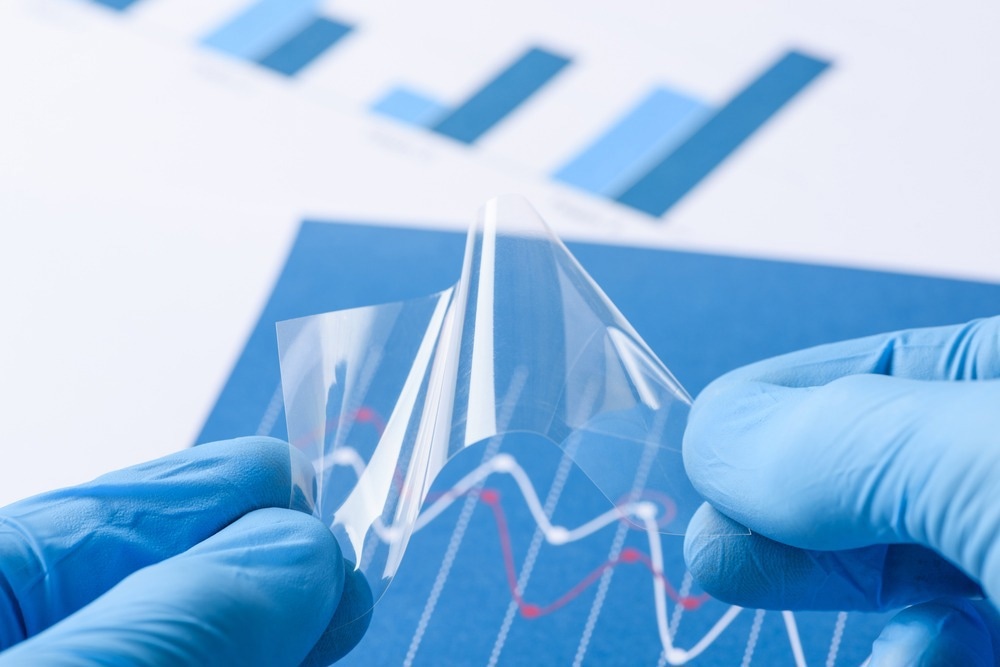
Image Credit: nevodka/Shutterstock.com
Plastics make up approximately 5–12 % of global waste generation, constituting around 20–30 % of waste by weight. Alarmingly, about 60 % of plastics end up as plastic waste (PW) in the environment.2
Conventional plastics are typically produced from fossil fuels like petroleum and natural gas, whereas biodegradable plastics are sourced from renewable materials such as plant and microbial biomass.
These renewable biopolymers exhibit chemical and mechanical characteristics comparable to those of traditional plastics.3 Biodegradable thin films, the most popular choice, contain additives that confer desirable properties and enable enzymes to break them down into simpler components decomposable by microorganisms.
Due to these advantageous characteristics, biodegradable thin films are widely chosen to promote net-zero emissions and significantly reduce plastic waste, thereby mitigating pollution.
An Introduction to Biodegradable Thin Films
Amid growing environmental concerns surrounding traditional plastic products, the world is turning to biodegradable thin film materials as an alternative. Biodegradable films and coatings, consisting of thin layers, are in high demand across various industries, particularly the food packaging industry.
These environmentally friendly packaging films and coatings primarily consist of proteins, polysaccharides, lipids, and antimicrobial ingredients. These coatings form a continuous thin layer, usually between 0.050 and 0.250 mm, which is deemed safe for consumption alongside food.4
The materials used to produce these films originate from renewable biological sources, predominantly starch, cellulose, hemicellulose, proteins, gelatin, lipids, fibers, and similar resources.
The applications of biodegradable thin films necessitate the incorporation of additives during manufacturing, such as antioxidants and antimicrobials, to enhance their structural strength and chemical stability.
Biodegradable plastics are decomposed into harmless elementary compounds by microorganisms in natural environments and conditions like soil, composting, anaerobic digestion, or water bodies. This results in the formation of harmless products, such as carbon dioxide, water, and methane.5
Compared to other degradation processes, biodegradation is significantly faster, and the by-products pose no harm to the environment.
Biodegradable thin films do not require special conditions or enzymes for breakdown and easily decompose alongside other organic waste. As a result, once their operational time is complete and they are discarded, there is no need to separate these thin films from other wastes.6 This saves time and money, making composting a sustainable and financially viable option.
Industries Leveraging Biodegradable Thin Films
Food packaging is a critical and intricate process, ensuring food quality according to standards while safeguarding it from germs and bacteria. Biodegradable films and coatings are unequivocally the foremost products for this purpose, providing the most sustainable packaging solutions.
Fresh produce, such as fruits and vegetables, have higher rates of respiration and transpiration, which can cause microbial spoilage. Active thin films offer a significant advantage by enabling manufacturers to maintain unique in-package atmosphere modification.
This allows for the maintenance of a tailored environment for different types of fruits, ensuring their freshness. PLA-based films have proven effective in controlling respiration rates and moisture loss, particularly in fresh-cut pineapples.7
In the dairy industry, there is a noticeable shift towards the use of biodegradable thin films. For instance, in 2011, a prominent German dairy company, Dannon, introduced PLA packaging for its Activia yogurt in Germany.
PLA packaging has also been adopted to store Danbo cheese in light and dark conditions. Additionally, antimicrobial biodegradable nisin films are utilized to inhibit yeast and mold growth, particularly in cheese products such as mozzarella.7
Biodegradable thin films show promise in addressing plastic debris accumulation and soil pollution. Active biodegradable films are considered the most effective alternative to traditional plastic films for winter rapeseed production.
In a study involving summer maize, researchers utilized a combination of three biodegradable films. Results showed a notable increase in soil temperature and water storage compared to traditional plastic film. The soil organic carbon content of the unaltered group was approximately 15 % higher in the group with biodegradable film, and these films also reduced the release of nitrogenous gases.8
In addition to the agriculture and packaging industries, biodegradable films are extensively used in the biomedical field. A prime example is chitosan-based biodegradable films in regenerative medicine, employed due to their biodegradability, porosity, and biomechanical compatibility.
Bone tissue engineering approaches using artificially fabricated grafts are very beneficial for the regeneration of both autograft and allograft bone. These films support bone growth at attachment sites and help maintain structural integrity during in
vivo tissue remodeling.9
These biodegradable films are also extensively employed for drug delivery in treating cancers, revolutionizing the biomedical field.
Challenges in Biodegradable Thin Film Technology
While biodegradability offers sustainability advantages, several challenges must be efficiently addressed. Biopolymers utilized in biodegradable thin films necessitate specialized industrial composting conditions for complete degradation, unlike backyard composting. This limits accessibility due to the limited availability of such facilities in many regions.
The biodegradation rates of thin active films can also vary significantly depending on the polymer type and environmental conditions, with reported timeframes ranging from less than 100 days to over a year.7
Experts have raised concerns about ecological toxicity originating from the accumulation of monomers, plasticizers, or nanoparticles released during fragmentation. Biodegradation also releases greenhouse gases like methane, which could exacerbate the impacts of climate change.7
In most areas, the lack of composting infrastructure and waste collection systems poses a significant challenge for the disposal of biodegradable plastics after use. These limitations threaten the commercialization of biodegradable thin films and strongly affect confidence in this technology.
Advancements in Biodegradable Thin Film Technology
Experts have begun using encapsulation technology to overcome the limitations of conventional biodegradable thin films and significantly boost their physiochemical stability. Encapsulation involves enclosing or coating one substance, whether in solid, liquid, or gaseous form, within another material known as the wall material.
This innovative technology aims to protect sensitive bioactive natural compounds from damage, particularly under harsh conditions.10 However, stringent European regulations concerning food contact materials and safety considerations have limited the application of numerous encapsulated antimicrobial and antioxidant agents in release systems.
Addressing legislative restrictions and establishing a unified global organization would facilitate the development of more precise and comprehensive regulations for materials in contact with food. This would enable greater specificity and accuracy in governing food-contact materials, thereby fostering innovation and ensuring food safety standards are met across borders.10
In another development, researchers have incorporated biodegradable star-shaped PCL-PDLA plasticizers to achieve super-tough yet highly biodegradable blends of Poly (lactide-co-glycolide) (PLGA).
These plasticizers were combined with PLGA to produce transparent thin films. With just a 0.5 wt % addition of star-shaped PCL-b-PDLA, the elongation at break of the PLGA blend increased to approximately 248 %, without significantly compromising its excellent mechanical strength or modulus.
The morphology of the PLGA blends revealed a robust cross-linked network between the PLLA and PDLA segments, which effectively enhanced interfacial adhesion.11
The Future of Biodegradable Thin Films
Various industries are now exploring biodegradable thin films due to their numerous advantages and benefits. The advancement of new biodegradable polymers necessitates simplicity, cost-effectiveness, and recyclability wherever feasible, offering significant employment opportunities. The emergence of new hybrid systems is anticipated, as these novel biodegradable polymers may exhibit effective and crucial properties.
More from AZoM: Laser Annealing for Crystalline Thin Films
References and Further Reading
[1] Paletta, A., et al. (2019). Barriers and challenges to plastics valorisation in the context of a circular economy: Case studies from Italy. Journal of Cleaner Production. doi.org/10.1016/j.jclepro.2019.118149
[2] Zhang, F., et al. (2021). Current technologies for plastic waste treatment: A review. Journal of Cleaner Production. doi.org/10.1016/j.jclepro.2020.124523
[3] Dirpan, A., et al. (2023). A Review on Biopolymer-Based Biodegradable Film for Food Packaging: Trends over the Last Decade and Future Research. Polymers. doi.org/10.3390/polym15132781
[4] Gupta, V., et al. (2022). A Comprehensive Review of Biodegradable Polymer-Based Films and Coatings and Their Food Packaging Applications. Materials. doi.org/10.3390/ma15175899
[5] Edaes, F., et al. (2020). Conventional Plastics' Harmful Effects and Biological and Molecular Strategies for Biodegradable Plastics' Production. Current Biotechnology. doi.org/10.2174/2211550109999201113102157
[6] Guo, C., et al. (2022). Progress in the Degradability of Biodegradable Film Materials for Packaging. Membranes. doi.org/10.3390/membranes12050500
[7] Cheng, J., et al. (2024). Applications of biodegradable materials in food packaging: A review. Alexandria Engineering Journal. doi.org/10.1016/j.aej.2024.01.080
[8] Sun, Y., et al. (2022). Past, present, and future perspectives of biodegradable films for soil: A 30-year systematic review. Frontiers in Bioengineering and Biotechnology. doi.org/10.3389/fbioe.2022.1006388
[9] Rezaei, F., et al. (2021). Chitosan films and scaffolds for regenerative medicine applications: A review. Carbohydrate polymers. doi.org/10.1016/j.carbpol.2021.118631
[10] Baghi, F., et al. (2022). Advancements in Biodegradable Active Films for Food Packaging: Effects of Nano/Microcapsule Incorporation. Foods. doi.org/10.3390/foods11050760
[11] Jeong J., et al. (2023). Super-Tough and Biodegradable Poly(lactide-co-glycolide) (PLGA) Transparent Thin Films Toughened by Star-Shaped PCL-b-PDLA Plasticizers. Polymers. doi.org/10.3390/polym1512261
Disclaimer: The views expressed here are those of the author expressed in their private capacity and do not necessarily represent the views of AZoM.com Limited T/A AZoNetwork the owner and operator of this website. This disclaimer forms part of the Terms and conditions of use of this website.