Mar 7 2016
When single crystals, especially silicon wafers, are fractured or broken up they pose a number of serious issues. The tip of the crack spreads at an atomic scale, resulting in chemical bonds breaking, the formation of crack fronts via the micrometer-scale crystal, and a culmination of severe wafer shattering on the macroscopic scale.
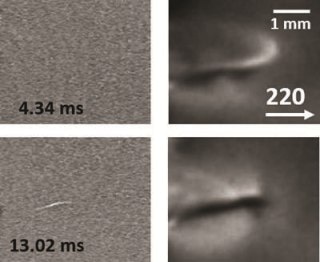
In the semiconductor industry the total breakage of wafers presents multi-scale issues during handling, as well as during temperature treatment processes. This costs a device manufacturing line millions and thousands of dollars every year.
Due to the high velocity inherent in the process, there is a lack of understanding about the applicable dynamics controlling an ideal cleavage along the {110} or {111} faces, and about the deflection into higher indexed faces of higher energy. Existing imaging methods come with certain limitations, because they merely display the initial state of wafers before the occurrence of a crack, and the final state of the wafers after the formation of cracks.
For the first time scientists have shown that this issue can be resolved by simultaneous imaging of in situ high-speed propagation of a crack, under thermal stress by using direct transmission and X-ray diffraction imaging techniques. A scientific commentary is also available on this (Tanner, 2016).
The researchers demonstrated how the atomic-scale propagation of crack tip and the associated strain field can be respectively monitored in the phase-contrast as well as diffracted images. Movies with microsecond time resolution for each frame show that the crack tip and strain do not spread at a steady speed.
Jumps occurring in the position of the crack tip denote restraining the crack tip for 1 to 2 ms, followed by jumps that are quicker than 2 to 6 ms-1. This leads to an average velocity of 0.028 to 0.055 ms-1 which is observed macroscopically. The results provide a proof of concept that the X-ray method can be used for studying ultra-speed cracks up to the velocity of sound.
We are only at the beginning of studying ultra-fast crack propagation in single-crystalline materials in real time.
Rack et al.