A team of researchers recently published a paper in the journal Scientific Reports that investigated the microstructure and printability of directed energy deposited 316 low carbon stainless steel (SS316L)-Inconel 718 (IN718) (SS316L-IN718) multi-material through experimental analysis and numerical modeling.
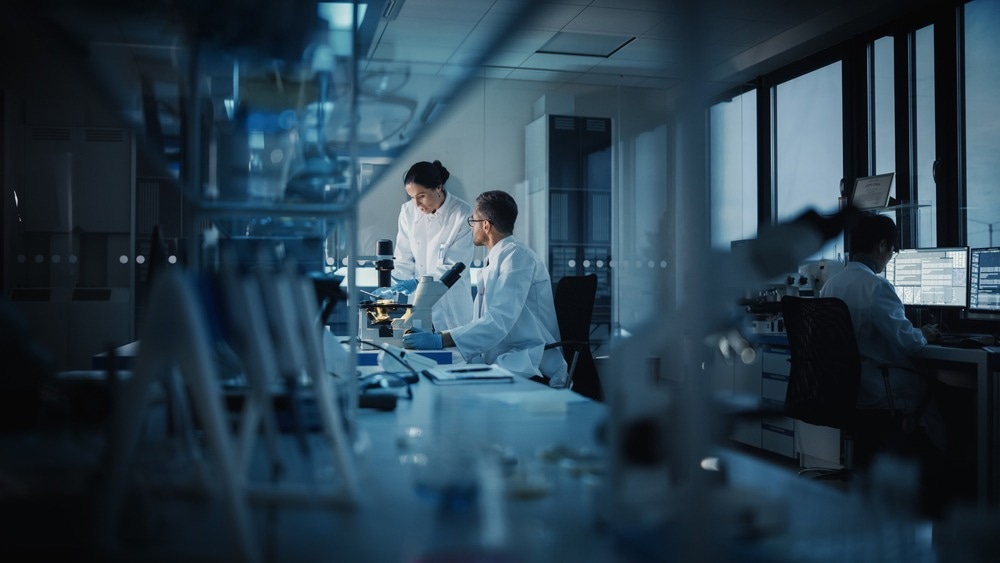
Study: Printability and microstructure of directed energy deposited SS316l-IN718 multi-material: numerical modeling and experimental analysis. Image Credit: Gorodenkoff/Shutterstock.com
Background
Multi-material structures play a crucial role in modern engineering sciences. The development of additive manufacturing (AM) technology has eliminated several limitations of traditional manufacturing methods and greatly facilitated the research and development of multi-materials.
AM technology possesses unique advantages, including high-level customization, cost-efficient small-scale production, and the single-step production of integrated near-net shape complex parts.
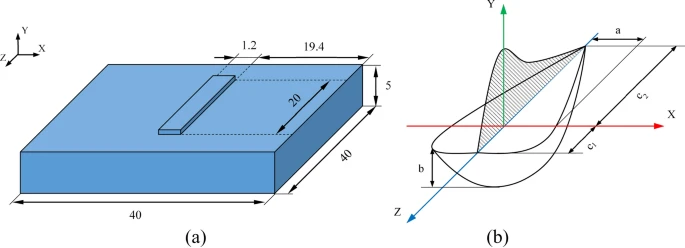
(a) The geometry of the single-layer deposition (mm). The deposition thickness was considered 0.8 mm according to the results obtained in experimental evaluations. (b) The double ellipsoidal heat source model and its parameters. Image Credit: Ghanavati, R et al., Scientific Reports
Metal AM processes, such as powder bed fusion (PBF) and directed energy deposition (DED), have gained significant popularity for multi-material fabrication. DED has received more attention compared to PBF owing to its greater flexibility in altering the chemical composition in the processing stage.
DED-processed metallic multi-materials can be classified as hybrid, functionally graded, and bimetallic materials based on the build strategy and nickel, iron, and titanium alloys based on the alloy type.
Stainless steel/nickel-based superalloy multi-materials are used extensively in critical energy industries as they can meet the service requirements by maintaining the cost-performance balance. In recent years, several studies have been performed on the AM of this type of multi-material.
Most of the studies have focused on the experimental evaluation of a single aspect of the materials paradigm/properties, microstructure, and AM of stainless steel/nickel-based superalloys multi-material.
However, a comprehensive understanding of all the interrelated aspects of microstructure-property-AM and the possibility of predicting them during the AM of multi-materials can lead to the development of an enhanced framework to control these aspects.
A numerical approach can assist in smoothly achieving the framework by reducing the cost and time required for different experiments.
The Study
In this study, researchers investigated the interrelated aspects of AM-microstructure-property in directed energy deposited SS316L-IN718 multi-material through numerical modeling and experimental evaluation.
They used the concept of printability through finite element modeling to evaluate the SS316L-IN718 multi-material processing challenges using the DED method. Researchers also used the solidification principles to investigate the characteristics of the microstructure and estimate multi-material properties. Experimental studies were performed to analyze and validate the numerical results.
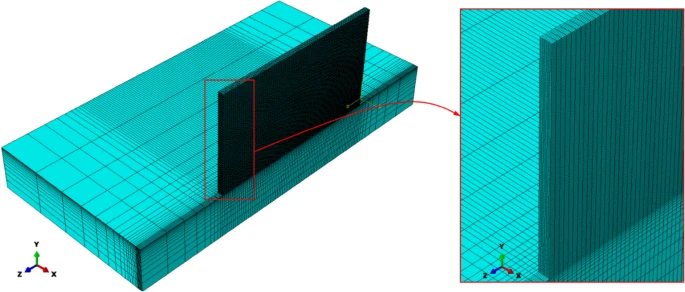
The mesh system used for the final model (14-layer structure). Image Credit: Ghanavati, R et al., Scientific Reports
Numerical Modeling
The finite element method (FEM) was used in numerical modeling for heat transfer modeling during the multi-material structure manufacturing process. The structure was composed of seven IN718 alloy layers and seven SS316L alloy layers.
The deposited layer surfaces were considered flat for simplification. Initially, the single-layer deposition geometry was modeled, and then the element birth and death technique was used to repeat the modeling procedure for the subsequent layers.
A double ellipsoidal power density distribution was used to model the laser heat source due to the necessity of experimental melt pool geometry observation in the cross-section and using the laser conduction mode during the AM process.
The governing heat transfer equation was solved using the ABAQUS v. 6.14 FEM software. A total of 226,820 nodes and 191,808 elements were utilized for the modeling. Additionally, the laser heat flux was applied as a function of time and location based on the double ellipsoidal distribution model using the ABAQUS user subroutine DFLUX.
Experimental Evaluation
IN718 and SS316L gas-atomized powders with 70 µm and 110 µm average diameters, respectively, and 40 × 40 × 5 mm SS316L substrate were used as raw materials.
A DED AM machine with a computerized numerical control (CNC) table with three degrees of freedom, shielding and carrier argon gas, twin powder feeder, four-channel nozzle delivering powder in conjunction with a laser beam, and a one kW continuous wave fiber laser with one mm spot diameter and 1080 nm wavelength was utilized to prepare the multi-material specimen.
The multi-material structure was prepared as a unidirectional thin wall composed of seven IN718 and SS316L layers. The thermal history of the multi-material structure fabrication was recorded using a K-type thermocouple embedded below and in the middle of the deposition path to validate the FE model.
Scanning electron microscopy (SEM) and optical microscopy were used to investigate the microstructure, while ImageJ software was used to quantify the microstructure characteristics. SEM with X-ray dispersive energy spectroscope was used for semi-quantitative evaluation of the microstructure. Additionally, a Vickers microhardness tester was used to measure the hardness variations in the build direction.
Observations
The multi-material printability analysis demonstrated that composition change defects and distortion increase with the increasing number of deposited layers owing to heat accumulation. However, a lack of fusion was observed in the initially deposited layers owing to efficient loss of heat through the SS316L substrate.
The IN718 section with 3.95 ×10−3 maximum thermal strain in the last layer was more sensitive to distortion owing to the difference in thermophysical properties of base materials that led to the formation of a larger melt pool.
The SS316L section with a more efficient heat loss in the initial layers and over 0.9% of manganese element vaporization in the sixth layer was more susceptible to lack of fusion and composition change.
Although a coarser solidification structure was formed with the progress of the DED process due to the decrease in cooling rate and increase in undercooling level, the eutectic reaction L→ gamma matrix phase (γ)+Laves intermetallic phase in the IN718 section led to the formation of finer dendritic microstructure in 8-14 layers compared to cellular microstructure in SS316L section.
The dendritic solidification of the IN718 section was separated from the cellular solidification of the SS316L section by reducing the temperature gradient/solidification rate ratio to almost 55 K s/mm2 and increasing the content of alloying elements with low distribution coefficients. However, an extremely narrow planar solidification mode was observed at the adjacent layer interface owing to the high local gradient temperature.
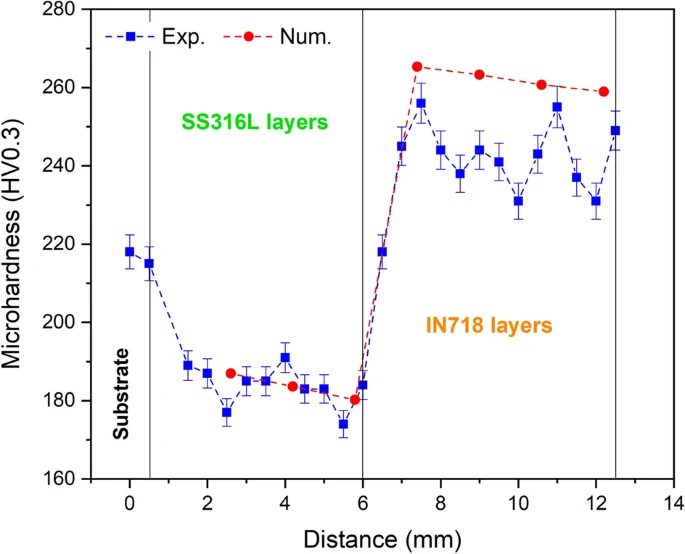
Microhardness variations along the cross-section of the multi-material structure. Image Credit: Ghanavati, R et al., Scientific Reports
The fraction of secondary phases, including Laves in IN718 and delta ferrite in SS316L, formed due to non-equilibrium solidification in the dendritic/intercellular regions increases linearly owing to cooling rate reduction in the multi-material build direction.
Moreover, the measurement and prediction of hardness in the build direction showed that the IN718 section possessed the highest hardness equivalent to 260 HV0.3 due to the existence of Laves intermetallic phase and enhanced yield strength of the matrix. However, variations in hardness were observed in every multi-material section with the rising number of layers owing to the decreasing secondary phase reinforcement and microstructure coarsening.
To summarize, this study comprehensively evaluated the interrelated aspects of AM-microstructure-property in directed energy deposited of SS316L-IN718 multi-material, which can assist in predicting these aspects during AM of this type and other types of multi-materials.
Disclaimer: The views expressed here are those of the author expressed in their private capacity and do not necessarily represent the views of AZoM.com Limited T/A AZoNetwork the owner and operator of this website. This disclaimer forms part of the Terms and conditions of use of this website.
Source:
Ghanavati, R., Naffakh-Moosavy, H., Moradi, M. et al. Printability and microstructure of directed energy deposited SS316l-IN718 multi-material: numerical modeling and experimental analysis. Scientific Reports 2022. https://www.nature.com/articles/s41598-022-21077-8