By Taha KhanReviewed by Lexie CornerApr 9 2024
Materials often exhibit distinct features and behaviors at different scales, necessitating a multi-scale approach to their characterization—from the atomic level to their macroscopic form. This comprehensive characterization reveals the intricate interplay between a material’s structure and properties, enabling a holistic understanding of its full potential and design for specific applications.1
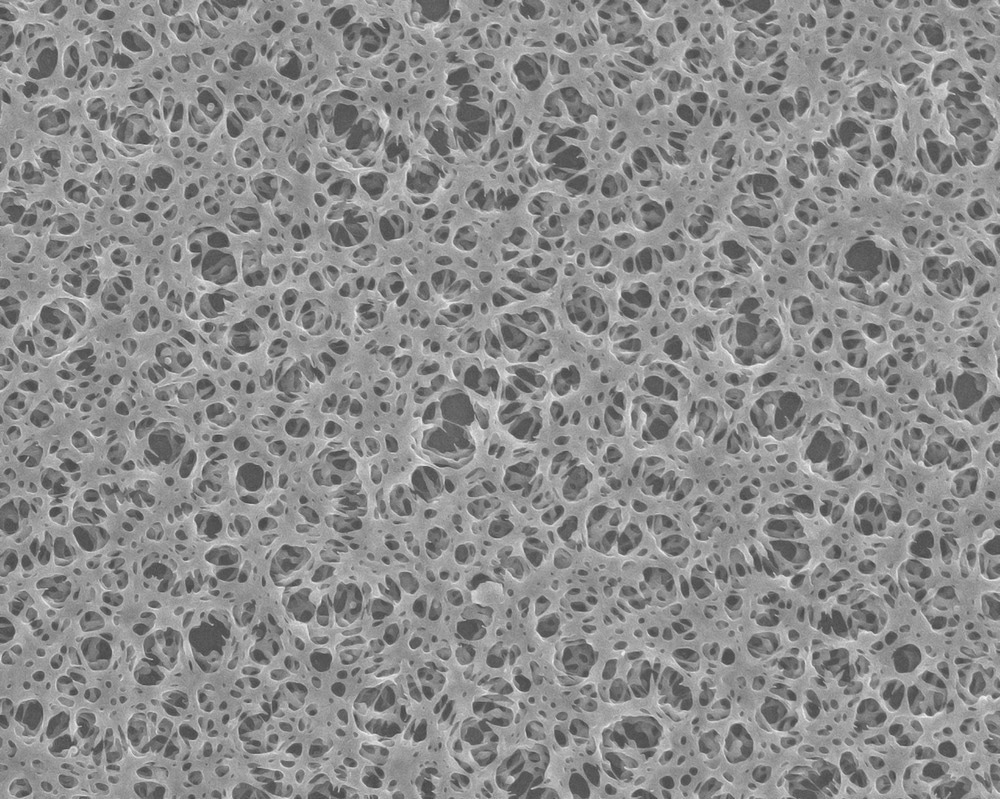
Image Credit: DooDee Studio/Shutterstock.com
Traditionally, analyzing materials involved techniques tailored for specific scales, which made integrating data from these different methods quite challenging. However, recent advancements in innovative material science technology, instrumentation, and computational approaches have helped bridge the gap between the micro and macroscales, thereby accelerating the field of materials characterization.1
This article discusses multi-scale materials characterization, highlighting recent innovations in characterization techniques and their impact on materials science and engineering.
What is a Multi-Scale Material?
Multi-scale materials are engineered or naturally occurring materials with distinct structural features and properties that span from the nanoscale (covering individual atoms or molecules to the microscale covering grains, pores, or inclusions) to the macroscale (where the material's bulk properties are observed). 2
The hierarchical structures inherent in multi-scale materials often contribute to their superior properties. For instance, composite materials with strong fibers embedded in a lighter matrix achieve exceptional strength-to-weight ratios.3,4
Similarly, nanomaterials such as graphene, renowned for their size-dependent properties, have significantly advanced electronics and energy storage applications. These materials have revolutionized several modern industries by providing specialized properties for various applications, ranging from lightweight modern airframes to intricate smartphone circuitry.
Evolution of Characterization Techniques
Traditionally, materials characterization relied on scale-specific techniques. However, these techniques were limited in their ability to bridge scales.
For instance, conventional optical microscopy provided valuable microstructural details, while mechanical testing revealed bulk properties. Similarly, electron microscopy offered exceptional resolution at the nanoscale but lacked the ability to capture the larger-scale organizational structures.5
Multi-scale characterization overcomes these limitations by combining diverse analytical techniques to create a comprehensive picture of a material.
This is achieved by using multiple microscopy platforms in tandem, such as correlative light and electron microscopy (CLEM), which allows for a seamless transition from broad structural observations to detailed nanoscale analyses.5
Cutting-Edge Multi-Scale Characterization Techniques
Multi-scale characterization continues to evolve thanks to novel technologies that utilize microscopy techniques, such as correlative light and electron microscopy and focused ion beam (FIB) milling. These methodologies offer unique advantages for the multi-scale characterization of materials.
For instance, FIB milling creates thin slices from a sample within a scanning electron microscope (SEM), enabling a smooth transition from large-scale imaging to high-resolution analysis of the internal structure.6
Similarly, computational quantum mechanical modeling methods, such as density functional theory (DFT), help scientists to simulate the behavior of materials at the atomic level. By integrating these simulations with multi-scale experimental data, researchers can explore the fundamental properties of materials, enabling the creation of more accurate models to predict their bulk behavior.7
Applications
These techniques have transformed several aspects of material science. For instance, in nanotechnology, multi-scale characterization helps understand the size-dependent properties of nanoparticles, crucial for optimizing their performance in applications like drug delivery and catalysis.
Similarly, in biomaterials research, these techniques allow scientists to mimic the hierarchical structure of natural tissues, enabling the development of more biocompatible implants.
Multi-scale characterization also helps optimize the interface between different components of advanced composites to develop stronger, lighter materials for aerospace applications.3,4
In a recent study, researchers investigated innovations in multi-scale materials characterization, focusing on extracellular vesicles (EVs) for therapeutic applications. By employing optical and non-optical techniques, they identified that the molecular composition of extracellular vesicles varied depending on the isolation method used, impacting their biological function.
The study introduced novel approaches, such as measuring extracellular vesicles' dry mass and predicting sedimentation, providing details of EV functionality.8
The researchers also explored the correlation between the physicochemical properties of EVs and their biological effects, emphasizing the significance of isolation methods for their application in diagnostics and therapeutics.
These findings are crucial for understanding the composition and functionality of EVs, offering a significant advancement in their clinical translation.8
Multi-scale characterization: Challenges and Future Solutions
Multi-scale characterization faces several challenges, including data integration, computational demands, and the need for advanced instrumentation.
Combining data from diverse techniques is complex. However, these challenges can be mitigated by standardizing data formats and developing robust data analysis pipelines.9
Similarly, the development of new instruments that offer higher resolution and sensitivity, along with the capability to examine materials under diverse in-situ conditions, is crucial.
The future of multi-scale materials characterization is promising, thanks to ongoing technological advancements. Emerging technologies, such as artificial intelligence and machine learning, hold immense potential for automating data analysis.10
Advancements in 3D printing and microfluidics could also help create in-situ characterization platforms. Such platforms will enable researchers to analyze materials under real-world operating conditions.11
Continued innovation in characterization techniques is vital for developing new materials and enhancing existing ones, underscoring its importance for the future of advanced materials characterization.
More from AZoM: Mass Spectrometry in the Characterization and Discovery of Metamaterials
References and Further Reading
- Lamberti, L. (2021). Advances in Multi-Scale Mechanical Characterization of Materials with Optical Methods. Materials. doi.org/10.3390/ma14237282
- Raabe, D., Scheffler, M., Kremer, K., Thiel, W., Neugebauer, J., Jansen, M. (2009). Multi-scale modeling in materials science and engineering. [Online] MPG. Available at: https://www.mpg.de/36458/cpt01
- Tam, LH., Wu, C., Yan, L., Lau, D. (2022). Multi-Scale Investigation on Fiber-Reinforced Composite Materials: From Structural Design, Property Characterization to Engineering Applications. Frontiers in Materials. doi.org/10.3389/fmats.2022.924213
- Zgłobicka, I., Kurzydłowski, KJ. (2022). Multi-length scale characterization of frustule showing highly hierarchal structure in the context of understanding their mechanical properties. Materials Today Communications. doi.org/10.1016/j.mtcomm.2022.104741
- Cognigni, F., Miraglia, L., Contessi, S., Biancardi, F., Rossi, M. (2023). Correlative Light and Electron Microscopy (CLEM): A Multifaceted Tool for the Study of Geological Specimens. Journal of Experimental and Theoretical Analyses. doi.org/10.3390/jeta1020006
- Mura, F., Cognigni, F., Ferroni, M., Morandi, V., Rossi, M. (2023). Advances in Focused Ion Beam Tomography for Three-Dimensional Characterization in Materials Science. Materials. doi.org/10.3390/ma16175808
- Ghosh, SK. (2003). Density functional theory and multiscale materials modeling. Bulletin of Materials Science. [Online] Indian Academy of Sciences. Available at: https://www.ias.ac.in/public/Volumes/boms/026/01/0003-0012.pdf
- Phan, TH., et al. (2021). New multiscale characterization methodology for effective determination of isolation–structure–function relationship of extracellular vesicles. Frontiers in Bioengineering and Biotechnology. doi.org/10.3389%2Ffbioe.2021.669537
- Ovchinnikova, OS. (2022). Correlated Materials Characterization via Multimodal Chemical Imaging and Data Analytics. Microscopy and Microanalysis. doi.org/10.1017/S1431927622004226
- Zhang, GX., Song, Y., Zhao, W., An, H., Wang, J. (2022). Machine learning-facilitated multiscale imaging for energy materials. Cell Reports Physical Science. doi.org/10.1016/j.xcrp.2022.101008
- Xia, Q., Sun, G., Kim, J., Li, Y. (2023). Multi-scale modeling and simulation of additive manufacturing based on fused deposition technique. Physics of Fluids. doi.org/10.1063/5.0141316
Disclaimer: The views expressed here are those of the author expressed in their private capacity and do not necessarily represent the views of AZoM.com Limited T/A AZoNetwork the owner and operator of this website. This disclaimer forms part of the Terms and conditions of use of this website.