By Surbhi JainReviewed by Susha Cheriyedath, M.Sc.Mar 23 2022
In an article recently published in the open-access journal Materials, researchers discussed the microstructural parameters which are essential for the modeling of superconducting foam.
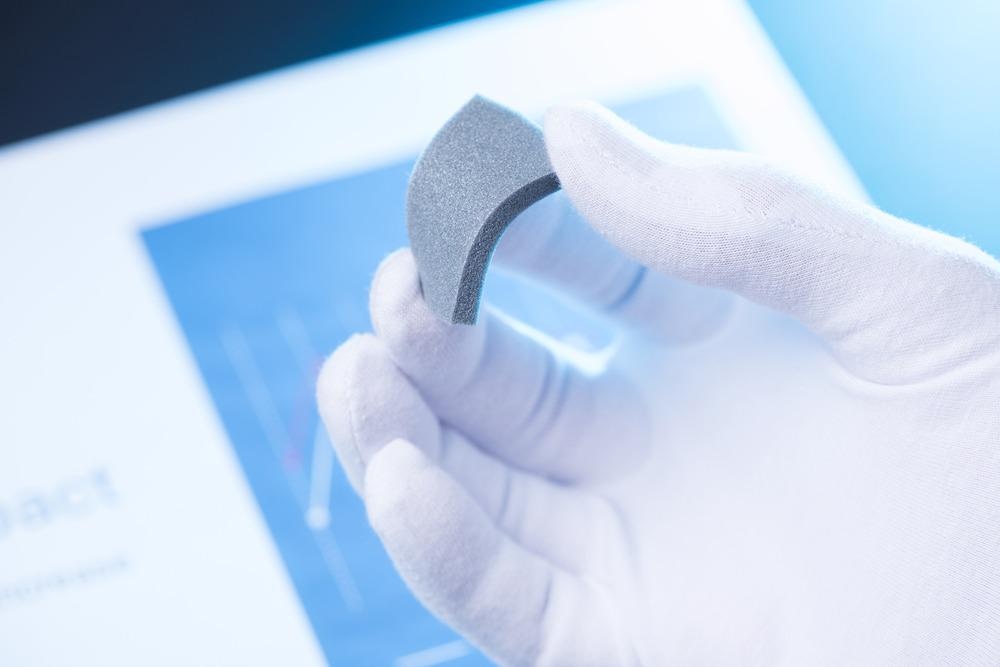
Study: Microstructural Parameters for Modelling of Superconducting Foams. Image Credit: nevodka/Shutterstock.com
Background
Due to their diverse, unique features, superconducting and open-cell YBa2Cu3Oy (YBCO) foam samples are fascinating materials for a number of applications. Trapped fields (TFs) measurements in superconducting YBCO foam samples indicated a more intricate TF pattern than in ordinary bulk samples.
The compression of native current loops in the sample was blamed for the existence of multiple tiny peaks in the TF patterns. These findings showed that the flow of current through a superconducting foam sample is complexed by the 3D configuration of the foam struts, allowing for the formation of local current loops.
Thus, a greater understanding of superconducting foam samples and their mechanical properties is required to enable their use in various applications. Modeling foam samples with a simple cell geometry and a regular succession of cells and as a first approximation has been shown to allow reasonable property prediction.
However, improving the model by utilizing factors from the real foam structure is important for a better demonstration of the mechanical properties of the polymer and metallic foam materials. As a result, it is critical to properly assess the microstructure of superconducting foams in order to obtain data for better modeling.
(a) Heat treatment to obtain the Y211 foam, (b) heat treatment (IG-process) converting the Y-211 foam to an superconducting YBCO foam. (c) Illustration of the foam samples at different process stages. Image Credit: Koblischka, M. R et al., Materials
About the Study
In this study, the authors discussed the utility of a Kelvin-type cell as a starting attempt for modeling the mechanical and superconducting properties of the foam samples. The results of a first modeling attempt in this direction were presented, along with experimental results at 77 K, for an estimation of the possible trapped fields (TFs). YBCO foam samples were examined using scanning electron microscopy, optical microscopy, and electron backscatter diffraction (EBSD) to provide a set of credible results.
The team determined a number of factors such as the shape and size of the windows and cells, the shape and length of the ligaments or foam struts, and the respective intersection angles to better represent the genuine foam structure. The foam microstructures were thoroughly analyzed to illustrate the difference between the original polymer foams and the superconducting foams fabricated through the infiltration growth (IG) process.
The authors examined the genuine foam microstructure of open-cell, superconducting YBCO foams to gain a better knowledge of the specifics of the foam microstructure. The structural factors crucial for modeling superconducting foam samples were also identified. The effects of the capillary flow of the liquid phase in the IG-process along the foam struts, the formation of Ba3Cu5Oy particles and flow patterns on the internal surfaces of the foam, and the formation of tiny Y-211 nanoparticles located in channel-like structures within the YBCO matrix were all revealed in a detailed analysis of the microstructure of superconducting YBCO foams.
SEM and digital optical microscopy were used to provide a better understanding of the real foam structure, as well as to determine significant changes from the polyurethane foams used as the basis material. The H-formulation and the regular Kelvin cell technique were used to model the magnetic characteristics and to reproduce the basics of the trapped field observations.
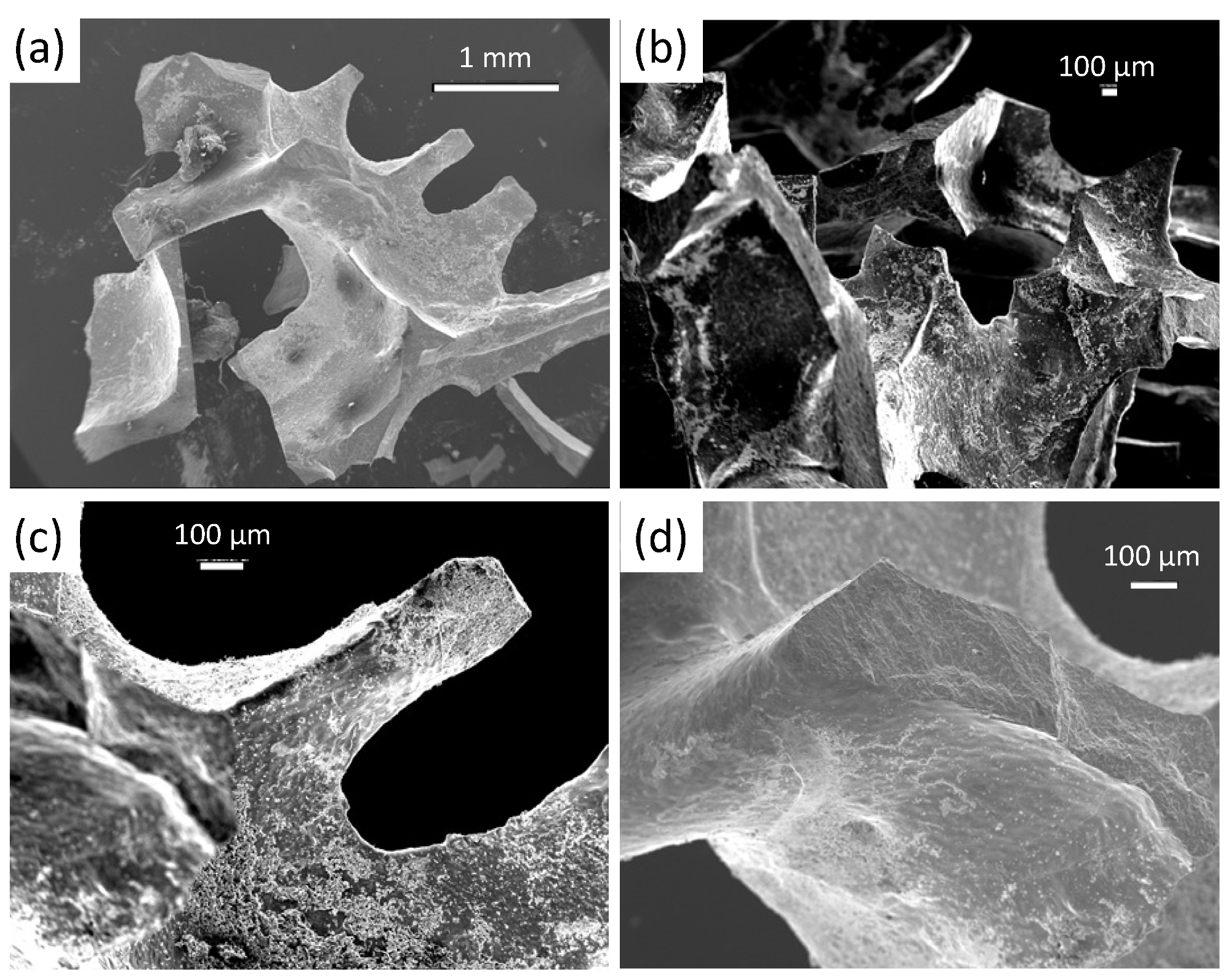
(a–d) SEM images at low magnification of broken-out foam strut pieces from a superconducting YBCO foam (magnification 25× (a), 35× (b), 85× (c) and 95× (d)), giving information of the real foam microstructure and the various internal surfaces existing in the open-cell foam structure. Note the rough character of the as-grown strut surfaces of the YBCO foam. The images further demonstrate the irregular shape and size distribution of the foam struts, which need to be modeled. Image Credit: Koblischka, M. R et al., Materials
Observations
The YBCO matrix was made up of elongated grains with orientations ranging from 30 to 60° off the [001]-orientation. The melt-textured samples had a density of 5.88 to 6.17 g/cm3, whereas the calculated density of YBCO was 6.4 g/cm3. Real foam cells were made up of irregular polyhedra with 9 to 17 faces. The mechanical properties of foams were found to be greatly influenced by the microstructure achieved and, as a result, by the fundamental qualities of the base material used to generate the foam sample.
On the strut surfaces, a high number of Ba3Cu5Oy particles with sizes ranging from 0.5 to 3 µm were scattered in an uneven pattern as individual particles or particle clusters. The EDX analysis revealed that the particles were mostly Ba3Cu5Oy, which was the pure liquid phase employed in the IG-processing. Aside from these particles, the SEM pictures showed unique growth steps and some distinctive patterns on the surfaces of struts, which were created by the liquid phase's capillary flow. EDX revealed that these patterns had a larger Y content than the particles on the surface.
Furthermore, the model results implied that a uniform distribution of cell sizes, rather than the irregular structure of the current YBCO foam material, provided greater TF performance.
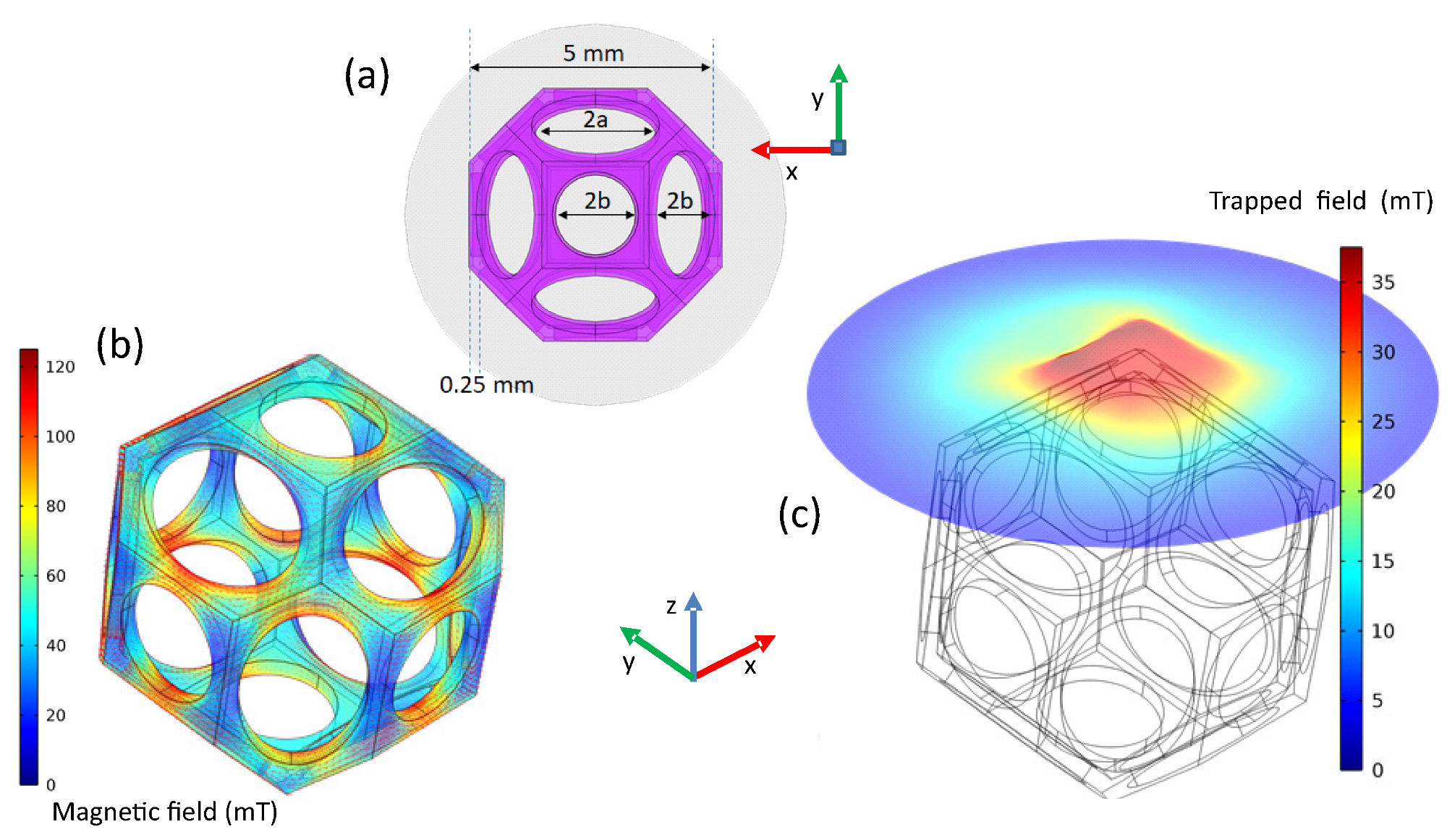
First modeling of trapped fields of a foam using the Kelvin cell, the dimensions of which are given in (a). (b) presents the distribution of the local magnetic field after field cooling in a field of 50 mT, and (c) gives the trapped field distribution at 2.75 mm above the foam. Image Credit: Koblischka, M. R et al., Materials
Conclusions
In conclusion, this study elucidated the differences between the original polymer foams used as the base material and the prepared superconducting YBCO foam samples. New information about the corresponding infiltration growth process was also presented which was attributed to a large amount of internal surface area in a foam sample.
The proposed simple modeling revealed useful information about the best foam structure for achieving large TF values. The authors emphasized the need for a refined model of a superconducting foam sample incorporating the real sample structure to determine various other parameters such as mechanical strength, magnetostriction, percolative current flow, and the details of the TF distribution. They also believe that a detailed description of the superconducting YBCO foams' microstructure is necessary.
Disclaimer: The views expressed here are those of the author expressed in their private capacity and do not necessarily represent the views of AZoM.com Limited T/A AZoNetwork the owner and operator of this website. This disclaimer forms part of the Terms and conditions of use of this website.
Source:
Koblischka, M. R., Koblischka-Veneva, A., Nouailhetas, Q., et al. Microstructural Parameters for Modelling of Superconducting Foams. Materials 15(6) 2303 (2022). https://www.mdpi.com/1996-1944/15/6/2303