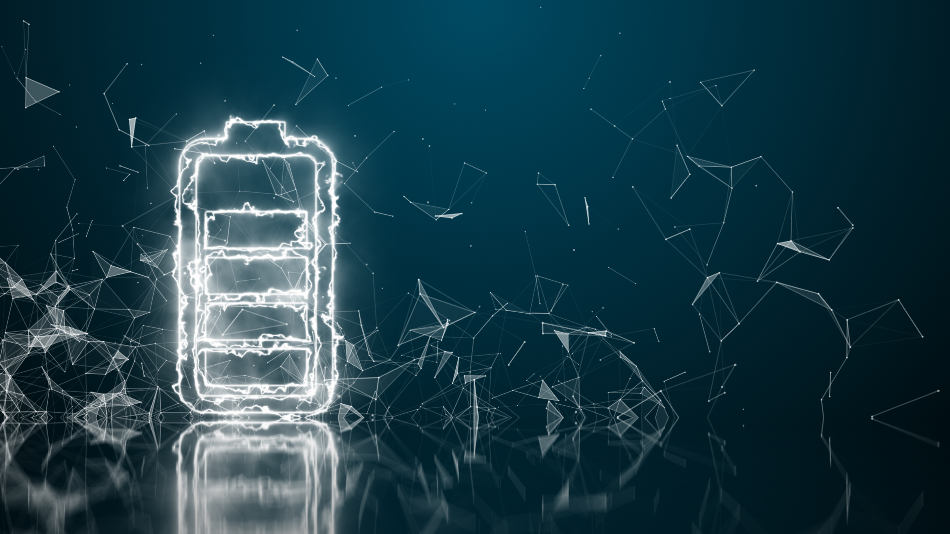
Image Credit: Immersion Imagery/Shutterstock.com
Today’s modern electronic devices are demanding greater energy storage to carry out increasingly complex tasks. They also need to be super flexible and durable without sacrificing performance. As the world of the Internet of Things continues to boom, we can expect the demand for devices such as wearables to continue to grow, all while their functions become more complex. As a result, scientists are exploring innovative ways to develop energy storage devices that have both high capacities but are also able to withstand bending and stretching. This has led to polymers becoming a key focus of these investigations.
However, not all polymers work alike, and it is essential to select the correct polymer for the electrodes and electrolytes used in these devices in order to achieve a device with the necessary functions. Below, polymer materials that research has determined suitable for use in energy storage devices will be discussed.
Polymer-Based Electrode Materials
Electrode materials that are used in the supercapacitors necessary for storage devices to function are one of two types. They are either electric double-layer capacitors (EDLCs) or are pseudocapacitive. EDLCs encompass most carbon-based materials, like active carbon, CNTs, graphene and its composites, and template carbon. The pseudocapacitive type, on the other hand, encompasses almost all metal oxides, such as conducting polymers, nitrides, sulfides, and their polymer composites.
The EDLC approach uses the physical electrostatic charge accumulation at the junction between the electrolyte and the electrochemically inert electrode materials to store energy, whereas the pseudocapacitive type uses fast surface redox reactions to store energy.
There are observable differences between the types of polymers used as electrode materials. Electrodes of the pseudocapacitive type are characterized by their high energy density in comparison to EDLCs, and their specific capacitance.
Polymer-Based Electrolytes
Electrolytes serve as a major component of electrochemical supercapacitors. It is vital for electrochemical performance due to its direct link with the device’s energy density. The electrolyte material’s electrochemically stable potential window (ESPW) limits the device’s cell voltage. In widening this ESPW of the electrolyte, the cell’s energy density can be improved upon.
Because of the decomposition potential for water, which is around 1.23 V in a neutral solution at room temperature, aqueous electrolytic supercapacitors usually work within 0 to 1.3 V. In contrast, electrolytes based on organic and ionic liquids are able to operate at a higher voltage range of 2.0 to 2.7 V and 3.5–4.0 V, respectively.
The result of this is that using organic or ionic liquid-base electrolytes in supercapacitors enables the device to obtain higher power and energy densities than those made with aqueous electrolytes.
In addition, the ions that exist within an electrolyte solution can also impact the efficiency of the electrochemical process.
Polymer-Based Multi-Functional Flexible Supercapacitors
Finally, due to the increasing demand for wearable devices, engineers are needing to develop memory storage devices with high energy storage capacities and high performance that are also lightweight, low density, flexible, and durable. Because of this, conductive polymers and porous carbon materials have become a major focus of these developments.
Additionally, the need for miniaturization of these devices to allow for their integration with multiple devices that have numerous functionalities has pressured researchers to investigate the development of multifunctional supercapacitors.
Finally, there is also a focus on designing and manufacturing flexible supercapacitors for use in wearables that do not pose adverse effects to the wearer, such as toxicity.
All these factors have impacted what polymers are suitable for use in energy storage devices. Recently, significant progress has been made in the creation of polymer-based multifunctional flexible supercapacitors. Below, the materials that have emerged as successful for the different applications of supercapacitors will be discussed.
To begin with, polymer hydrogels that behave as stretchable electrolytes in combination with stretchable substrates have become relied upon for the development of stretchable supercapacitors.
For devices that use self-healable supercapacitors, polymer-based supercapacitors have been developed that rely on the abundant hydrogen bonds between the polymer hydrogels to give them their self-healing characteristics.
Supercapacitors that require shape memory are constructed from polymers via one of two separate approaches. Either polymers with shape memory properties are applied to a fabricated electrode material, or shape memory alloy wires are used as the device’s shape-memory substrate.
Finally, for electrochromic supercapacitors, conducting polymer-based materials are being developed to create flexible and wearable electrochromic devices that are also multifunctional. The fabrication of these polymer-based electrochromic supercapacitors allows for simultaneous energy storage and energy-sensing via color change by charge insertion, extraction, or electrochemical oxidation/reduction.
Further Research into Capacitor Construction
In addition to the work being done on polymer materials appropriate for next-generation storage devices, research is also taking place that is exploring innovative ways to construct capacitors that enable greater storage capacities.
For example, a research project conducted at the Queen Mary University of London was inspired by the structure of croissants to develop a new dielectric capacitor built from pressing and folding a polymer film capacitor. This resulted in a dielectric capacitor with the ability to store 30 times more energy than others that are commercially available.
Soon, we can expect further research to advance our knowledge of how to use polymers in energy devices to enhance storage as well as to give them the new properties required by modern devices. In addition, we can also expect research exploring how these capacitors should be structured to enhance storage capacity.
References and Further Reading
Isah, S. (2018). Advanced Materials for Energy Storage Devices (Review). Asian Journal of Nanoscience and Materials, 1(2). http://www.ajnanomat.com/article_61451.html
Meng, N., Ren, X., Santagiuliana, G., Ventura, L., Zhang, H., Wu, J., Yan, H., Reece, M., and Bilotti, E. (2019). Ultrahigh β-phase content poly(vinylidene fluoride) with relaxor-like ferroelectricity for high energy density capacitors. Nature Communications, 10(1). https://www.nature.com/articles/s41467-019-12391-3
Wang, Z., Zhu, M., Pei, Z., Xue, Q., Li, H., Huang, Y. and Zhi, C. (2020). Polymers for supercapacitors: Boosting the development of the flexible and wearable energy storage. Materials Science and Engineering: R: Reports, 139, p.100520. https://www.sciencedirect.com/science/article/pii/S0927796X19301135?via%3Dihub#sec0150
Disclaimer: The views expressed here are those of the author expressed in their private capacity and do not necessarily represent the views of AZoM.com Limited T/A AZoNetwork the owner and operator of this website. This disclaimer forms part of the Terms and conditions of use of this website.